

Evolution and the Diversity of Life
Selected Essays
Harvard University Press books are not shipped directly to India due to regional distribution arrangements. Buy from your local bookstore, Amazon.co.in, or Flipkart.com.
This book is not shipped directly to country due to regional distribution arrangements.
Pre-order for this book isn't available yet on our website.
This book is currently out of stock.
Edit shipping location
Dropdown items
- Barnes and Noble
- Bookshop.org
- Waterstones
ISBN 9780674271050
Publication date: 03/25/1997
The diversity of living forms and the unity of evolutionary processes are themes that have permeated the research and writing of Ernst Mayr, a Grand Master of evolutionary biology. The essays collected here are among his most valuable and durable: contributions that form the basis for much of the contemporary understanding of evolutionary biology.
Mayr has shown himself to be a true follower of Darwin, Wallace, and Huxley. His writings are lively and critical, and they provide remarkable insight into the development of the field. —Peter H. Raven, Science
The range of topics covered in these essays is awesome when one realizes that they are the product of a single mind. —Francisco J. Ayala, Sciences
Ernst Mayr not only ranks among the great evolutionary biologists of this century; he is also one of the best writers. This collection is of lasting importance. —E. O. Wilson
- Ernst Mayr was Alexander Agassiz Professor of Zoology, Emeritus, at Harvard University. He was the recipient of numerous honorary degrees and awards, including the Crafoord Prize for Biology, the National Medal of Science, the Balzan Prize, and the Japan Prize.
Book Details
- 6-1/8 x 9-1/4 inches
- Belknap Press
From this author

Systematics and the Origin of Species from the Viewpoint of a Zoologist

This Is Biology

The Evolutionary Synthesis

One Long Argument

Toward a New Philosophy of Biology
Recommendations.

Inheritance

Good Enough

Adaptive Oncogenesis

Chimpanzees and Human Evolution
Sorry, there was an error adding the item to your shopping bag.
Expired session
Sorry, your session has expired. Please refresh your browser's tab.
Main navigation
An item has been added to the cart
Added to shopping bag
- Copy to clipboard
Set your location
It looks like you're in   . Would you like to update your location?
Unavailable
Harvard University Press titles are not shipped directly to India due to local distribution arrangements.
Unavailable in country .
Shopping Bag
Your shopping bag is currently empty. Add items to your shopping bag, to complete check out.
Academia.edu no longer supports Internet Explorer.
To browse Academia.edu and the wider internet faster and more securely, please take a few seconds to upgrade your browser .
Enter the email address you signed up with and we'll email you a reset link.
- We're Hiring!
- Help Center
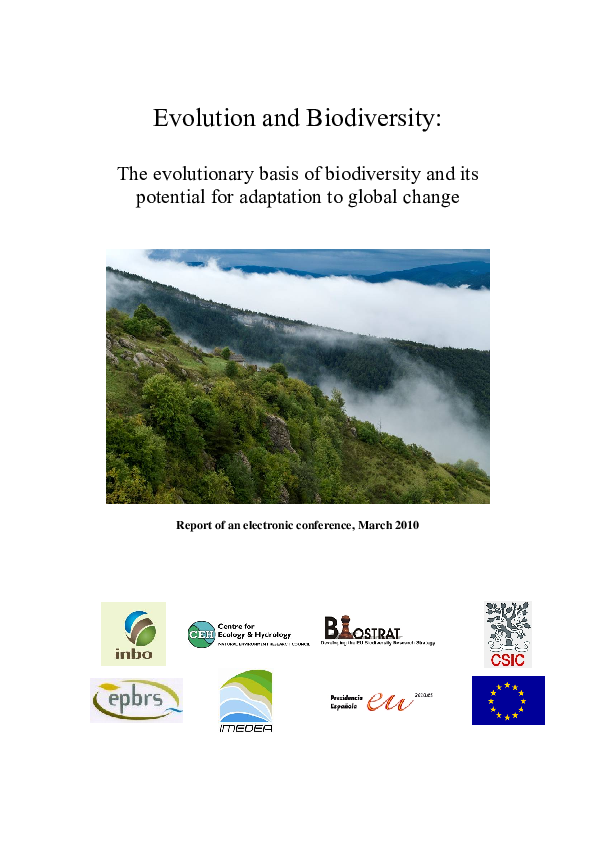
Evolution and biodiversity: the evolutionary basis of biodiversity and its potential for adaptation to global change

Page 1. Evolution and Biodiversity: The evolutionary basis of biodiversity and its potential for adaptation to global change Report of an electronic conference, March 2010 Page 2.
Related Papers
Andrew Hendry
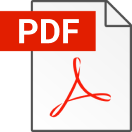
The Environmentalist
Nixon Ayala
A large proportion of existing species — possibly half, conceivably even more — may be lost within the foreseeable future. But this may not prove to be the most consequential outcome of the current biodiversity crisis. More significant could be the disruption and degradation of several basic processes of evolution. It appears likely that for mass extinction episodes (MEEs) in the geological past, the recovery period usually lasted at least five million years. Because of certain unique features of the present MEE — notably the near elimination of biomes such as tropical forests, wetlands and coral reefs, which have served as ‘powerhouses’ of evolution in the past — the ‘bounce-back’ phase could extend several times longer than five million years. Among distinctive features of future evolution could be; in the short term, homogenization of biotas, a proliferation of opportunistic species, an outburst of speciation among particular taxa, and a pest-and-weed ecology; and, in the long term, a decline of biodisparity, the elimination of megavertebrates, an end to speciation among large vertebrates, and multiple constraints on origination, innovation and adaptive radiation. These disruptive phenomena would rank among the most prominent departures in the entire course of evolution. Full knowledge and understanding of what may characterize future evolution remains largely a black hole of research. As a consequence, conservation policies fail to reflect a further problem of the biodiversity prospect, perhaps exceeding the better recognized problem of the mass extinction of species.
Journal of Biosciences
R. Geeta , Andrew Hendry
ABSTRACT Biodiversity is a key to human well-being. However universally acknowledged, this reality is not appre-ciated as much as one might imagine or hope. Having achieved a high standing in the Convention on Biological Diversity (CBD) in 1992, biodiversity appears to have gradually fallen from that position in the 20 years since. This is evidenced by the fact that most discussions and actions in relation to the CBD have now shifted to sustainable development and ecosystem services (Daily 1997), instead of focusing more directly on biodiversity values themselves. One reason for this fall in status seems to be linked to issues about the actual definition of 'biodiversity', whether used in a broad sense, including the diversity of genes, species, communities and ecosystems, or more narrowly defined as the diversity of species alone. While the close link between biodiversity and 'ecosystem services' is undeniable, biodiversity itself has long recognized links to human well-being, including 'option values' (see later) that are sometimes neglected (Faith 2012). The conservation of biodiversity and all its values is fundamental to strategies aimed at achieving the long-term goal of sustainable development. The CBD definition of biodiversity captures the importance of considering variation and its values at multiple levels, including genetic and trait variation (http://www.cbd.int/convention/articles/default.shtml?a=cbd-02). A model that is explicitly evolutionary in nature provides the most coherent and powerful descriptor of biodiversity, and yet evolutionary thinking is generally neglected in most plans to manage our environment. It is critical, however, that an evolutionary perspective be incorporated into conservation planning (Hendry et al. 2010). 1. Evolution generates and maintains biodiversity and ecosystems Biodiversity is a product of evolution and, in the broadest sense, includes all biological variation on Earth, present and past. Organisms, the relationships among them, and the relationships between organisms and their abiotic environment are constantly changed by evolution. Given this, it might seem obvious that the principles of evolutionary biology should have a major role in regional planning for land or water uses as well as for managing ecosystems. Thus, an understanding of evolutionary processes necessarily comple-ments current emphasis on their ecological counterparts. It is easy to acknowledge that 'nothing in biology makes sense except in the light of evolution'. However, it is necessary to go beyond the generality of this statement and find ways to apply our understanding of evolutionary processes to the conservation and management of ecosystems (e.g. Sarkar and Margules 2002). 2. Incorporating evolution in the valuation of biodiversity for sustainable use
Molecular Phylogenetics and Evolution
Régis Ferrière
Population viability is determined by the interplay of environmental influences and individual phenotypic traits that shape life histories and behavior. Only a few years ago the common wisdom in evolutionary ecology was that adaptive evolution would optimize a population’s phenotypic state in the sense of maximizing some
Luis Santamaria
The intensity and speed of human alterations to the planet’s ecosystems are yielding our static, ahistorical view of biodiversity obsolete. Human actions frequently trigger fast evolutionary responses, affect extant genetic variation and result in the establishment of new communities and co-evolutionary networks for which we lack past analogues. Contemporary evolution interplays with ecological changes to determine the response of organisms and ecosystems to anthropogenic pressures. Examples on wild species include responses to harvest (e.g. fisheries, hunting, angling), habitat loss and fragmentation (e.g. genetic effects of isolation), biotic exchange (e.g. evolutionary responses to control measures), climate change (e.g. local adaptation and its interplay with dispersal processes) and the responses of endangered species to conservation measures. A review of international and EU biodiversity policies showed numerous opportunities for the integration of evolutionary knowledge, with the realistic prospect of improving their efficacy. Such opportunities should be extended to other sectoral policies of direct relevance for biodiversity – notably nature conservation, fisheries, agriculture, water resources, spatial planning and climate change. These avenues for improvement are, however, challenged by the low level of enforcement of biodiversity policies, linked to the nonbinding nature of most biodiversity-policy documents, and the decreasing representation of biodiversity in EU’s research policy.
Elisabeth Huber-Sannwald
Integrative and Comparative Biology
Robert K Colwell
Journal of the History of Biology
John Beatty
Stefan Porembski
RELATED PAPERS
New Phytologist
Guy Midgley
Phoebe Barnard
I. Swingland
Laurie Furlong
Paola Migliorini , Marcellof Buiatti M Buiatti
Global Environmental Change
Pim Martens
James Cook University eBooks
Petina Pert
CBD Technical Series No. 54
Terence Hay-Edie
European Journal of ecology
Rogério Martins
Douglas Riff
Francine Kershaw
J. Chris Pires
Philosophical Transactions of The Royal Society B: Biological Sciences
Evolution: Education and Outreach
Daniel Brooks
Yueh-hsin Lo
Michel Loreau
Evolutionary Conservation Biology
Luke Harmon
Annual Review of Ecology Evolution and Systematics
Sébastien Lavergne
Molecular ecology
Andy Purvis
Journal of Agriculture and Sustainability
Barbara Sawicka , Dickson Adom
Emmanuel Fara , Arnaud Brayard
mirna kurnia
Craig Hilton-Taylor
- We're Hiring!
- Help Center
- Find new research papers in:
- Health Sciences
- Earth Sciences
- Cognitive Science
- Mathematics
- Computer Science
- Academia ©2024
ENCYCLOPEDIC ENTRY
Biodiversity.
Biodiversity refers to the variety of living species on Earth, including plants, animals, bacteria and fungi. While Earth’s biodiversity is so rich that many species have yet to be discovered, many species are being threatened with extinction due to human activities, putting the Earth’s magnificent biodiversity at risk.
Biology, Ecology
grasshoppers
Although all of these insects have a similar structure and may be genetic cousins, the beautiful variety of colors, shapes, camouflage, and sizes showcase the level of diversity possible even within a closely-related group of species.
Photograph by Frans Lanting
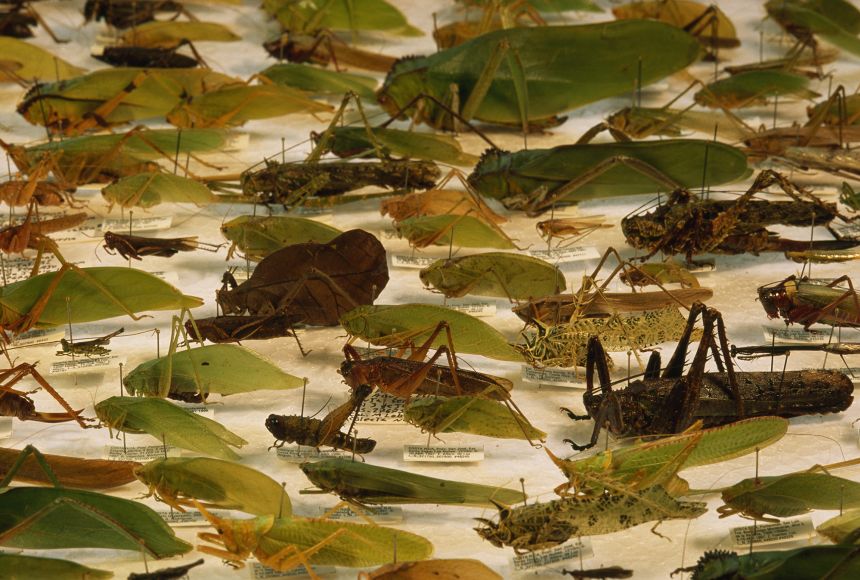
Biodiversity refers to all the different kinds of living organisms within a given area, including plants, animals, fungi and other living things. It includes everything from towering redwood trees to tiny, single-cell algae that are impossible to see without a microscope.
Kinds of Biodiversity
A common way to measure biodiversity is to count the total number of species living within a particular area. Tropical regions—areas that are warm year-round—have the most biodiversity. Temperate regions, which have warm summers and cool winters, have less biodiversity. Regions with cold or dry conditions, such as mountaintops and deserts, have even less biodiversity.
Generally, the closer a region is to the Equator, the greater the biodiversity. At least 40,000 different plant species live in the Amazon Rainforest of South America, one of the most biologically diverse regions on the planet. By contrast, only around 600 plant species live on the Antarctic continent and in its lakes.
The warm waters of the western Pacific and Indian Oceans tend to have the most diverse marine environments. The Bird’s Head Seascape in Indonesia is home to more than 1,600 species of fish and more than 70 percent of the world’s coral species. Many of the corals build coral reefs, which are home to hundreds more species, from tiny seaweeds to large sharks.
Some regions in the world have a large number of endemic species, meaning species that exist only in that place. The Cape Floristic Region in South Africa is home to about 6,200 plant species found nowhere else in the world. Areas with high numbers of endemic species are called biodiversity hotspots. Scientists and communities are making a special effort to preserve biodiversity in these regions.
Biodiversity can also refer to the variety of ecosystems, or communities of living things and their environments. Ecosystems include deserts, grasslands and rainforests, among others. The African continent is home to tropical rain forests, alpine mountains and dry deserts. It enjoys a high level of biodiversity. Antarctica, covered almost entirely by an ice sheet, has low biodiversity.
Another way to measure biodiversity is through genetic diversity. Genes are the basic units of biological information that are passed on when living things reproduce. Human beings have about 25,000 genes. Some genes are the same for all individuals within a species—they are what make a daisy a daisy and a dog a dog. But some genes within members of the same species are different. This genetic variation is why some dogs are poodles and some are pit bulls, or why some people have brown eyes and some people have blue eyes.
Greater genetic diversity within a species can make that species more resistant to diseases, and it allows a species to better adapt to a changing environment.
Importance of Biodiversity
All species are interconnected; they depend on one another. Forests provide homes for animals. Animals eat plants, which need healthy soil to grow. Fungi help decompose organisms to fertilize the soil. Bees and other insects carry pollen from one plant to another, which enables the plants to reproduce. With less biodiversity, these connections weaken, and sometimes break, harming all the species in the ecosystem.
Ecosystems with a lot of biodiversity are generally stronger and more resistant to disease than those with fewer species. For instance, some diseases kill only one kind of tree. In the early 1900s, American chestnut blight killed most of the chestnut trees in the eastern forests of North America. The forest ecosystem was able to survived because other kinds of trees also grew there.
Biodiversity is important to people in many ways. Plants, for instance, help humans by giving off oxygen. They also provide food, shade, construction material, medicines and fiber for clothing and paper. The root system of plants helps prevent flooding. Plants, fungi and animals, such as worms, keep soil fertile and water clean. As biodiversity decreases, these systems break down.
Hundreds of industries rely on plant biodiversity. Agriculture, construction, medical and pharmaceutical, and fashion industries all depend on plants for their success. When the biodiversity of an ecosystem is interrupted or destroyed, the economic impact on the local community can be enormous.
Biodiversity is especially important to the medical and pharmaceutical industries. Scientists have discovered many chemicals in rainforest plants that are now used in helpful medications. One of the most popular and safe pain relievers, aspirin, was originally made from the bark of willow trees.
Medicines that treat some forms of cancer have been made from rosy periwinkle ( Catharanthus roseus ), a flower that grows on the African island of Madagascar. Traditional medicine practitioners across Africa and Asia have used the flower for centuries to treat diabetes and stomach issues. This led scientists to investigate the chemistry of the plant in the 1950s, leading to the discovery that it could be used to treat cancer.
Indigenous people around the world, particularly those who live in tropical rainforests, have long used plants for medicinal purposes. Scientists have studied only a small percentage of rainforest species in their search for cures. Many important drugs have evolved from field observations of how Indigenous people use certain plants for healing. This kind of research is called ethnobotany. But thousands of species are at risk of becoming extinct before scientists can determine whether they might be useful in medicines.
Decreasing Biodiversity
In the past hundred years, biodiversity around the world has decreased dramatically. Thousands of species are in danger of extinction. Extinction is a natural process; some species naturally die out while new species evolve. But human activity has changed the natural processes of extinction and evolution. Scientists estimate that species are dying out at hundreds of times the natural rate.
Human activity altering and destroying natural habitats is a major reason for the loss of biodiversity. As habitats shrink, fewer species can live there. Those that survive have fewer breeding partners, leading to a decline in genetic diversity.
People and corporations clear and pollute land to plant crops, keep livestock, further develop cities, mine precious metals or build housing and factories. Human-driven climate change and development have destroyed about 35% of the world’s wetlands since 1970, a habitat that 40% of plant and animal species depend on. People cut down forests for lumber and to make space for cattle ranching, which accounts for 80% of deforestation in Amazon countries. Companies have cleared large areas of rainforest in Indonesia and Malaysia to create vast plantations of palm oil trees. Companies use the fruit of the tree to create palm oil for commercial use. This large-scale deforestation is destroying habitats for critically endangered wildlife.
Pollution, overfishing and overhunting have also caused a drop in biodiversity. Global climate change—the latest rise in the average temperature around the globe, linked to human activity—is also a factor. Warmer ocean temperatures damage fragile ecosystems, such as coral reefs. A single coral reef can shelter thousands of fish species and other sea creatures, such as clams and sea stars. Scientific research has linked climate change to human activities, like burning fossil fuels and clearing forests for agriculture.
Biodiversity can also be harmed by non-native species. When people introduce species from one part of the world to another, they typically have no natural predators. These non-native species thrive in their new habitat, often destroying native species in the process. Brown tree snakes, for instance, were accidentally brought into Guam, an island in the South Pacific, in the 1950s. Because brown tree snakes have no natural predators on Guam, they quickly multiplied. The snakes, which prey on birds, have hunted at least 10 of the island’s native forest-dwelling bird species to extinction.
People all over the world are working to maintain the planet’s biodiversity. In the United States, the Endangered Species Act protects over 2,000 organisms that are in danger of extinction. In a historic move in 2021, African Parks relocated 30 white rhinos from South Africa to Rwanda’s Akagera National Park to establish a protected breeding population of white rhinos. Some organizations are working to create sustainably grown mushrooms to satisfy consumers as well as the local ecosystem.
Around the globe, thousands of wilderness areas have been set up to conserve plants, animals and ecosystems. Local, national and international organizations are cooperating to preserve the biodiversity of regions threatened by development or natural disasters. The United Nations Educational, Scientific and Cultural Organization (UNESCO) World Heritage Site program recognizes areas of global importance, such as the enormous wetland region of the Pantanal in South America. Many national parks, such as Glacier National Park in the U.S. state of Montana, protect biodiversity within the park by restricting extractive activities, such as mining and drilling. In Ecuador, the Jocotoco Foundation has purchased land across the country to protect the habitats of threatened wildlife. More than 16 biological reserves have been established, including areas in the Amazon rainforest and the Galapagos Islands.
Marine protected areas (MPAs) have been established to preserve sea life. In the MPA around Australia’s Great Barrier Reef, no-fishing zones have helped fish populations thrive. People are also working to limit pollution and restore coral reef ecosystems in the area. As ecosystems become healthier, their biodiversity increases.
Media Credits
The audio, illustrations, photos, and videos are credited beneath the media asset, except for promotional images, which generally link to another page that contains the media credit. The Rights Holder for media is the person or group credited.
Production Managers
Program specialists, last updated.
August 9, 2024
User Permissions
For information on user permissions, please read our Terms of Service. If you have questions about how to cite anything on our website in your project or classroom presentation, please contact your teacher. They will best know the preferred format. When you reach out to them, you will need the page title, URL, and the date you accessed the resource.
If a media asset is downloadable, a download button appears in the corner of the media viewer. If no button appears, you cannot download or save the media.
Text on this page is printable and can be used according to our Terms of Service .
Interactives
Any interactives on this page can only be played while you are visiting our website. You cannot download interactives.
Related Resources
If you're seeing this message, it means we're having trouble loading external resources on our website.
If you're behind a web filter, please make sure that the domains *.kastatic.org and *.kasandbox.org are unblocked.
To log in and use all the features of Khan Academy, please enable JavaScript in your browser.
AP®︎/College Biology
Course: ap®︎/college biology > unit 7.
- Introduction to evolution and natural selection
- Natural selection and the owl butterfly
- Biodiversity and natural selection
- Variation in a species
Darwin, evolution, & natural selection
Natural selection.

Key points:
- Charles Darwin was a British naturalist who proposed the theory of biological evolution by natural selection.
- Darwin defined evolution as "descent with modification," the idea that species change over time, give rise to new species, and share a common ancestor.
- The mechanism that Darwin proposed for evolution is natural selection . Because resources are limited in nature, organisms with heritable traits that favor survival and reproduction will tend to leave more offspring than their peers, causing the traits to increase in frequency over generations.
- Natural selection causes populations to become adapted , or increasingly well-suited, to their environments over time. Natural selection depends on the environment and requires existing heritable variation in a group.
What is evolution?
Early ideas about evolution, influences on darwin, darwin and the voyage of the beagle.
- Traits are often heritable. In living organisms, many characteristics are inherited, or passed from parent to offspring. (Darwin knew this was the case, even though he did not know that traits were inherited via genes.)
- More offspring are produced than can survive. Organisms are capable of producing more offspring than their environments can support. Thus, there is competition for limited resources in each generation.
- Offspring vary in their heritable traits. The offspring in any generation will be slightly different from one another in their traits (color, size, shape, etc.), and many of these features will be heritable.
- In a population, some individuals will have inherited traits that help them survive and reproduce (given the conditions of the environment, such as the predators and food sources present). The individuals with the helpful traits will leave more offspring in the next generation than their peers, since the traits make them more effective at surviving and reproducing.
- Because the helpful traits are heritable, and because organisms with these traits leave more offspring, the traits will tend to become more common (present in a larger fraction of the population) in the next generation.
- Over generations, the population will become adapted to its environment (as individuals with traits helpful in that environment have consistently greater reproductive success than their peers).
Example: How natural selection can work
Key points about natural selection, natural selection depends on the environment, natural selection acts on existing heritable variation, heritable variation comes from random mutations, natural selection and the evolution of species, attribution:, works cited:.
- Wilkin, D. and Akre, B. (2016, March 23). Influences on Darwin - Advanced. In CK-12 biology advanced concepts . Retrieved from http://www.ck12.org/book/CK-12-Biology-Advanced-Concepts/section/10.18/ .
- Reece, J. B., Urry, L. A., Cain, M. L., Wasserman, S. A., Minorsky, P. V., and Jackson, R. B. (2011). The voyage of the Beagle . In Campbell Biology (10th ed., p. 466). San Francisco, CA: Pearson.
- Darwin's finches. (2016, April 25). Retrieved March 16, 2016 from Wikipedia: https://en.wikipedia.org/wiki/Darwin%27s_finches .
- Reece, J. B., Urry, L. A., Cain, M. L., Wasserman, S. A., Minorsky, P. V., and Jackson, R. B. (2011). Figure 1.18. Natural selection. In Campbell biology (10th ed., p. 14). San Francisco, CA: Pearson.
Additional references:
Want to join the conversation.
- Upvote Button navigates to signup page
- Downvote Button navigates to signup page
- Flag Button navigates to signup page

Essay on Biodiversity for Students and Children
500+ words essay on biodiversity.
Essay on Biodiversity – Biodiversity is the presence of different species of plants and animals on the earth. Moreover, it is also called biological diversity as it is related to the variety of species of flora and fauna. Biodiversity plays a major role in maintaining the balance of the earth.

Furthermore, everything depends upon the biological diversity of different plants and animals. But due to some reasons, biodiversity is decreasing day by day. If it does not stop then our earth could no longer be a place to live in. Therefore different measures help in increasing the biodiversity of the earth.
Methods to Increase Biodiversity
Building wildlife corridors- This means to build connections between wildlife spaces. In other words, many animals are incapable to cross huge barriers. Therefore they are no able to migrate the barrier and breed. So different engineering techniques can make wildlife corridors. Also, help animals to move from one place to the other.
Set up gardens- Setting up gardens in the houses is the easiest way to increase biodiversity. You can grow different types of plants and animals in the yard or even in the balcony. Further, this would help in increasing the amount of fresh air in the house.
Get the huge list of more than 500 Essay Topics and Ideas
Protected areas- protected areas like wildlife sanctuaries and zoo conserve biodiversity. For instance, they maintain the natural habitat of plants and animals. Furthermore, these places are away from any human civilization. Therefore the ecosystem is well maintained which makes it a perfect breeding ground for flora and fauna. In our country, their various wildlife sanctuaries are build that is today spread over a vast area. Moreover, these areas are the only reason some of the animal species are not getting extinct. Therefore the protected areas should increase all over the globe.
Re-wilding – Re-wilding is necessary to avert the damage that has been taking place over centuries. Furthermore, the meaning of re-wilding is introducing the endangered species in the areas where it is extinct. Over the past years, by various human activities like hunting and cutting down of trees the biodiversity is in danger. So we must take the necessary steps to conserve our wildlife and different species of plants.
Importance of Biodiversity
Biodiversity is extremely important to maintain the ecological system. Most Noteworthy many species of plants and animals are dependent on each other.
Therefore if one of them gets extinct, the others will start getting endangered too. Moreover, it is important for humans too because our survival depends on plants and animals. For instance, the human needs food to survive which we get from plants. If the earth does not give us a favorable environment then we cannot grow any crops. As a result, it will no longer be possible for us to sustain on this planet.
Biodiversity in flora and fauna is the need of the hour. Therefore we should take various countermeasures to stop the reduction of endangering of species. Furthermore, pollution from vehicles should decrease. So that animals can get fresh air to breathe. Moreover, it will also decrease global warming which is the major cause of the extinction of the species.
Customize your course in 30 seconds
Which class are you in.

- Travelling Essay
- Picnic Essay
- Our Country Essay
- My Parents Essay
- Essay on Favourite Personality
- Essay on Memorable Day of My Life
- Essay on Knowledge is Power
- Essay on Gurpurab
- Essay on My Favourite Season
- Essay on Types of Sports
Leave a Reply Cancel reply
Your email address will not be published. Required fields are marked *
Download the App
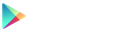
Warning: The NCBI web site requires JavaScript to function. more...

An official website of the United States government
The .gov means it's official. Federal government websites often end in .gov or .mil. Before sharing sensitive information, make sure you're on a federal government site.
The site is secure. The https:// ensures that you are connecting to the official website and that any information you provide is encrypted and transmitted securely.
- Publications
- Account settings
- Browse Titles
NCBI Bookshelf. A service of the National Library of Medicine, National Institutes of Health.
National Research Council (US) Committee on Research Opportunities in Biology. Opportunities in Biology. Washington (DC): National Academies Press (US); 1989.

Opportunities in Biology.
- Hardcopy Version at National Academies Press
8 Evolution and Diversity
Evolution and diversity result from the interactions between organisms and their environments and the consequences of these interactions over long periods of time. Organisms continually adapt to their environments, and the diversity of environments that exists promotes a diversity of organisms adapted to them. In recent years, new techniques and approaches have opened exciting new avenues of investigation of the processes that generate evolution and diversity. As a result, greater opportunities exist now for advancing knowledge than during any period since the 1930s and 1940s, when evolutionary biology and genetics became united in what came to be called the modem synthesis of evolutionary biology.
- The Processes and Results of Evolution Are Exemplified in the Evolution of Insecticide Resistance in Insects and Antibiotic Resistance in Bacteria
The first synthetic organic insecticide to be adopted for practical use was DDT, which was introduced in 1941. DDT appeared to have many advantages because, in proper dose, it was toxic to insects but not to humans. As a consequence, DDT was quickly employed worldwide to control houseflies, mosquitoes, and a variety of other insect pests. After the initial success of DDT, many other exotic chemical compounds were introduced as insecticides. The introduction and widespread use of each of these was quickly followed by the evolution of resistance in large numbers of insect species. In fact, more than 200 species of insects had become resistant to DDT by 1976; some species have evolved multiple resistance to four or more groups of chemical insecticides.
In many cases, the insecticide resistance results from the action of a single gene, although multiple other genetic changes that can modify the response to insecticides also occur. In the common housefly, resistance results from the presence of an enzyme called DDTase, the natural function of Which is unknown. Mutant forms of the enzyme convert DDT into the relatively harmless compound DDE. Resistance in the mosquito Aedes aegypyti is also associated with a DDTase enzyme, but not the one found in the housefly.
The evolution of resistance to insecticides is so common because the insect populations often contain rare mutant variants that are already resistant. Exposure to the insecticide gives an advantage to these mutants, and over several generations, they gradually increase in frequency at the expense of the normal types until very few of the normal sensitive types remain.
A remarkable principle in population genetics states that insecticide resistance can be expected to evolve in approximately 5 to 50 pest generations, irrespective of the insect species, geographical region, nature of the pesticide, frequency and method of application, and other seemingly important variables. The phenomenon occurs because the time required to evolve significant resistance depends on the logarithm of the total increase in frequency of the resistance gene as a result of the pesticide application, which over a wide range of realistic values is effectively limited to 5 to 50 generations. The rapid, repeated evolution of insecticide resistance in many parts of the world reflects the operation of this simple mathematical principle.
A similar situation accounts for the repeated evolution of antibiotic resistance in bacteria: Rare bacterial types containing genes for resistance are favored in the presence of the antibiotic and eventually displace the normal sensitive types. In this case, the overuse of inexpensive antibiotics, not only in medicine but in animal feed, fish culture, and agriculture, has promoted the evolution of antibiotic resistance in a wide spectrum of microorganisms. In many cases, the resistance genes are contained in mobile genetic elements that can be transmitted from one organism to the next, and their spread has resulted in the wide dissemination of the resistance genes among pathogenic and nonpathogenic forms.
The molecular evolution of antibiotic resistance is similar to the process that bacteria have used for millenia to evolve resistance to naturally occurring antibiotics and to soil contaminated with lethal concentrations of heavy metals. A resistance gene that evolves in one bacterial species can potentially be disseminated to many others by means of molecules known as plasmids, which are transmitted among suitable hosts by cell contact. These plasmids occasionally pick up transposable DNA sequences that contain genes resistant to antibiotics, and they confer resistance upon host cells. When the antibiotics are widely used and present in the environment, cells containing the resistance plasmids are favored, and the plasmid spreads. In many cases resistance plasmids have acquired genes for simultaneous resistance to five or more chemically unrelated antibiotics. For some pathogenic bacteria, such as gonorrhea, antibiotic resistance has become so widespread that clinical treatment is severely compromised.
The evolution of insecticide resistance in insect populations, antibiotic resistance in microbial populations, herbicide resistance in plant populations, and heavy-metal tolerance in plant and bacterial populations has been demonstrated repeatedly. In every case, genetic variation and natural selection provide an amazingly effective process for promoting the adaptation of organisms to their environments. The study of evolution and diversity of life on earth is concerned with the tempo, mode, and patterns of such adaptations.
EVOLUTION OF INSECTICIDE RESISTANCE
Some of the most dramatic examples of evolution in action result from the natural selection for chemical pesticide resistance in natural populations of insects and other agricultural pests. In the 1940s, when chemical pesticides were first applied on a large scale, an estimated 7 percent of the agricultural crops in the United States were lost to insects. Initial successes in chemical pest management were followed by a gradual loss of effectiveness. Today, more than 400 pest species have evolved significant resistance to one or more pesticides, and 13 percent of the crop of yields in the United States is lost to insects.
In many cases, significant pesticide resistance has evolved in 5 to 50 generations in spite of great variation in the insect species, the insecticide, and the method of application. Theoretical population genetics helps us understand this apparent paradox. Many of the insecticide resistances result from single mutant genes. The resistance genes are often partially dominant, so the change in the frequency of the resistance gene is governed approximately by the equation
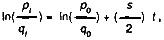
in which p and q are, respectively, the gene frequencies of the resistant and sensitive genes, initially (time 0) and at time t generations after insecticide application, and s measures the degree to which resistant insects are favored over sensitive ones.
Prior to application of the pesticide, the gene frequency p 0 of the resistant mutation is generally close to 0. Application of the pesticide increases the gene frequency, sometimes by many orders of magnitude, but significant resistance is noticed in the pest population even before the gene frequency p 1 increases above a few percent. Thus, as rough approximations, we may assume that q 0 and q 1 are both close enough to 1 that In( p 0 / q 0 ) = In( p 0 ) and In( p 1 / q 1 ) = In( p 1 ). Using these approximations, the equation implies that t = (2/ s ) In( p 1 / p 0 ). In many cases, the ratio p 1 / p 0 may range from 1 × 10 2 to perhaps 1 × 10 7 , and s may typically be 0.5 or greater. Over this wide range of parameter values, time t is effectively limited to 5 to 50 generations for the appearance of a significant degree of pesticide resistance. Details in actual cases will depend on such factors as the size of the insect population and the extent of genetic isolation between local populations, and the evolution of polygenic resistance may be expected to take somewhat longer than single-gene resistance. Nevertheless, the example demonstrates the predictive power of mathematical approaches in evolutionary biology.
- Some of the Most Exciting Current Research Opportunities in Evolution and Diversity Result from Technical Innovations in Molecular Biology
The techniques of molecular biology have revealed a rich level of detail in studies of DNA variation and its analysis. They have uncovered an unexpected avenue of genomic evolution through the activities of transposable elements. They have opened up the transfer of individual genes between species as a major new tool for the study of the mechanisms and subsequent events in speciation. And they have made possible an integration of the techniques of molecular biology with questions of field natural history, such as in the use of mitochondrial DNA polymorphisms to study population structure and migration in fish and other organisms.
Application of the techniques of molecular biology has made possible, for the first time, the beginnings of a synthesis of microbiology and evolutionary biology. These two fields have developed in almost complete isolation from each other. Microbiology is among the least evolutionarily oriented of biological disciplines, and evolutionary biology is the evolutionary biology of metazoans. Studies at the interface of these disciplines will result in the definition of new evolutionary principles and a deeper understanding of principles already established. Perhaps the most surprising initial result of studies in microbial evolution has been the discovery of what some scientists regard as a distinct kingdom of organisms, the archaebacteria, which combine some features of more familiar kinds of bacteria (eubacteria) with others characteristic of eukaryotic organisms.
Indeed, paleobiologists now believe that the earth's biota was composed solely of bacteria for at least two-thirds of its total history. Many evolutionary innovations have been powered by changes in intracellular biochemistry rather than by changes in the shape, size, or physical organization of organisms. Moreover, the global biota, especially bacteria, with their diverse physiological capabilities, have interacted with and changed the global environment in numerous crucial ways, such as in the creation of our oxygen-rich atmosphere.
The application of molecular techniques has also contributed to the current revolution in systematics. Molecular studies of DNA and proteins are now used routinely to distinguish species and to estimate phylogenetic relations among closely related species. Direct DNA sequencing is providing phylogenetically useful data almost faster than they can be analyzed. The inferred genealogical relations based on macromolecules are usually consistent with those based on morphology, but molecular studies often help to resolve relations that are morphologically ambiguous.
Overall similarity in macromolecules provides a reliable measure of evolutionary time only when the molecules being studied evolve at much the same rate in different lineages and at different times. Whether DNA sequences actually evolve with regular rates like molecular clocks is still much debated, but the data so far suggest at least moderate regularity. The concept of the molecular clock has provided a unique and powerful time dimension in evolutionary studies and has augmented as well as complemented inferences from the fossil record. However, not all of the evidence is consistent with the hypothesis that molecular evolution occurs at a nearly constant rate, and further evidence is needed to establish the validity of the hypothesis and to determine its range of application.
The explosive increase in knowledge of DNA sequences has created an acute need for new kinds of computational technologies and algorithms, as well as new statistical approaches, so that the data can be interpreted to maximum advantage. Appropriately analyzed, the new kinds of data will reveal, with a level of detail never before possible, patterns in the history of evolution; the new data will thus shed light not only on the evolution of macromolecules, but also on the processes of evolution of morphology, life histories, and physiology. Regrettably, the analysis of sequence data, which must bring together experts in statistics, computer science, mathematics, molecular biology, population genetics, developmental biology, and systematics, has lagged behind as ever more data have accumulated. At the same time, more extensive data on the extent of DNA sequence variation within species are badly needed.
- Technical Innovations That Have Transformed Studies of Evolution and Diversity Are Not Limited to Molecular Biology
Studies in biomechanics, ecology, and behavior have profited tremendously from improved techniques of photography and telemetry, and in almost every area of study, modern computers facilitate sophisticated simulation modeling and data analysis. Paleobiology has benefited from methods of organic geochemistry that enable the determination of the nature and isotopic characteristics of biologically derived organic compounds preserved in ancient sediments and also from new techniques of radiometric age determination and new data regarding the geologic and plate-tectonic history of the earth. These approaches, when combined with information derived from molecular biology, promise to promote new knowledge about such fundamental evolutionary events as the origin of invertebrates, vertebrates, plants, and human beings. Even earlier Precambrian events, such as the advent of photosynthesis, oxygen-dependent respiration, nucleated cells, eukaryotic sexual reproduction, and the modern type of anaerobic-aerobic global environment, may be within the reach of the new approaches.
Progress in the study of evolution and diversity does not require technical innovations, although it frequently benefits from them. Advances also come from the synthesis of previously disconnected areas, from new ways of looking at problems, or from new concepts. Therefore, in evolution and diversity, too much stress on technical virtuosity and trendiness runs the risk of promoting a kind of brush-fire pattern of scientific advance, with great activity and excitement near the front but little behind in the area where the practical applications of basic discoveries are often developed.
Although many exciting directions in evolution and diversity have been opened by advances in molecular biology, numerous fundamental problems occur at levels of biological organization above that of molecules. The evolution of populations of organisms is affected by the interactions with the environment of physiology, development, and behavior at levels that are not amenable to molecular analysis. Molecular biology is an aid but not a panacea in the discovery and classification of organisms. And the processes of speciation and extinction, while fundamental to evolution and diversity, are population, not molecular, processes.
- The Evolutionary Process
Population Genetics Continues to Emphasize Genetic Variation — Its Nature, Causes, and Maintenance in Populations
Studies of population genetics or genetic variation have become significantly more sophisticated with the use of molecular techniques and new types of material, including microbial organisms and chloroplast and mitochondrial DNA. Progress in molecular biology has been especially helpful for population genetics and promises to aid in the resolution of several outstanding problems in the field. Genetic variation can be resolved at the ultimate level of the DNA sequence. With this level of resolution, it becomes possible to determine whether genes that are highly variable within populations also evolve rapidly. The distribution of DNA polymorphisms within and among species results from the operation of evolutionary forces that are in many cases too weak or too difficult to measure in the laboratory or field; it may be possible to infer their magnitude from analysis of the sequences themselves. The rich possibilities of inferences that may be made from DNA sequence data warrant significant efforts in this direction.
The Technique of Site-Directed Mutagenesis Also Opens New Possibilities for Population Genetics
Traditionally, inferences about evolutionary constraints on molecular structure have been gathered from comparisons of homologous molecules among species. With site-directed mutagenesis, the inferences can be tested directly by deliberately altering parts of the molecule of interest, reintroducing the gene for the altered molecule into living organisms, and studying the effects of the changes. Such experiments reveal not only which changes affect the molecule, but also the magnitude of these effects. For the first time, population geneticists are able to study a collection of mutant molecules that are well characterized at the molecular level.
DNA SEQUENCES IN EVOLUTIONARY STUDIES
Comparisons of DNA and protein sequences have revolutionized the reconstruction of the evolutionary relations among organisms because the sequences themselves contain information about their ancestral history that can be extracted by appropriate statistical methods. Equally powerful inferences can be drawn from comparisons of sequences among individuals within species. This is possible because the sequences also contain information about the evolutionary forces that molded them, which can be studied to make inferences about the magnitude of natural selection, the importance of random processes, the role of recombination, and so on.
Two studies of DNA sequences among natural isolates of the bacterium Escherichia coli underscore the power of the molecular approach. One study focused on DNA sequences in the gnd gene, which codes for the enzyme 6-Phosphogluconate dehydrogenase. The purpose was to estimate the fraction of observed amino acid polymorphisms that are selectively neutral. This has been a central issue in population genetics for more than a decade, but it has defied resolution because most statistical tests of observed gene frequencies and most laboratory experiments lack sufficient power to detect selection coefficients of the relevant magnitude. Although most random amino acid substitutions might be expected to be harmful, only a small proportion of harmful mutations ever become established as polymorphisms in natural populations. A significant proportion of alleles that become polymorphic might therefore be expected to be selectively or nearly neutral.
The idea behind the study of DNA sequences is that nucleotide polymorphisms at silent sites, which do not change amino acid sequences, can be used as internal standards for comparison with amino acid polymorphisms in the same gene. When 768 nucleotides in the gnd gene in seven strains of E. coli were compared, 12 amino acid polymorphisms and 78 silent polymorphisms were found. All 12 amino acid polymorphisms occurred in singleton configurations (meaning that six strains shared a common amino acid at the site and only one strain was different), whereas only about half of the silent polymorphisms exhibited this configuration. Based on this difference alone, one can conclude the no more than six of the amino acid substitutions could be selectively neutral. Alternatively, if all amino acid polymorphisms are assumed to be mildly harmful, the amount of selection necessary to account for the preponderance of singleton configurations is only about 1.6 × 10 -7 , an amount of selection much too small to detect except by means of DNA sequence comparisons.
The second study concerned the occurrence of genetic recombination among natural isolates of E. coli . Evidence for recombination was found in a region of 1,871 nucleotide pairs around the phoA gene, which codes for alkaline phosphatase, in 10 natural isolates. The region contained 87 polymorphic nucleotide sites, of which 42 were shared by two or more strains. Comparisons of the shared polymorphisms gave clear evidence for intragenic recombination in that polymorphic nucleotides common to two or more strains tended to be spatially clustered within the gene. The putative exchange events involved short stretches of DNA on the order of several hundred nucleotide pairs. Although reproduction in E. coli is thought to be largely clonal, clonal reproduction is nevertheless consistent with recombination involving short stretches of DNA because most genes are still transmitted uniparentally. This is yet another example of how a DNA sequence can contain information about its history that cannot easily be inferred from direct experiments.
The process of mutation, which until recently seemed to result from an essentially simple process of nucleotide substitution or rearrangement, is now appreciated to include mechanisms for creating evolutionary novelty through the movement and other activities of transposable elements. Indeed, virtually all proteins may have been created in evolution by the rearrangement of exon units, which code for smaller structural domains able to fold autonomously and carry out elementary functions such as ligand binding. If true, this would mean that the evolution of new functions cannot be likened to the proverbial monkey pecking away at a typewriter in hope of creating something meaningful; the analogy should rather be to a monkey that can shuffle complete words and entire sentences and paragraphs.
Recombination, traditionally viewed as important from the standpoint of creating genetic variation through new combinations of genes, has taken on a new dimension in population genetics because of its conservative role in maintaining similarity between members of multigene families. However, little is known about the rate of gene conversion in multigene families or about the role of intragenic recombination in creating new genetic variation.
The Study of Natural Selection Remains One of the Principal Preoccupations of Evolutionary Biologists
The understanding of the mechanisms of selection in natural populations is still inadequate. At the molecular level, it is necessary to understand how changes in protein molecules affect fitness and to critically evaluate the contribution of selectively neutral mutations to molecular evolution. These problems are ideal for the application of site-directed mutagenesis in experimental organisms such as bacteria, yeast, and Drosophila . At the phenotypic level, it is necessary to understand how genes affecting quantitative characters respond to natural selection. This is an area in which substantial advances in the theory have been made recently and in which further progress can be expected. Analysis of multifactorial traits is essential to understanding the genetic basis and inheritance of many genetically complex disease traits in humans, including the most common birth defects and adult disorders. It is also important in evolution and diversity in interpreting the evolution of such multifactorial traits as morphology.
Significant methodological problems in natural selection include difficulties in measuring reproductive components, including fertility selection and sexual asymmetry in selection, nuclear-cytoplasmic gene interactions in fitness, and the elaboration of statistical models and experimental designs to estimate fitness components when there is inbreeding (as in some plants). Studies of selection in natural habitats are often hampered by lack of a rigorous, quantitative approach to studying the environment and its variation.
Evaluation of the role of population structure in evolution is also marred by important unresolved problems, such as the need to improve methods of estimating migration rate, to define the role of interactions between genotypes in selection, and to evaluate the significance of selection among demes (a local population of closely related organisms) in the genetic divergence and transformation of populations. Genetic differentiation results in variation among populations, and methods for the statistical analysis of such spatial patterning are now being developed.
Progress Is Being Made in Our Understanding of Speciation and the Evolution and Maintenance of Diversity
Organismal diversity is a direct and inevitable outcome of speciation, the process whereby a single species evolves into two or more distinct ones. The conditions required to initiate, promote, and complete the speciation process are still poorly understood and hence controversial. To resolve this problem, a major effort has been made in recent years to examine the biological and genetic attributes of closely related taxa actively undergoing various degrees of differentiation. Three approaches are taken in these investigations: field, experimental, and theoretical.
Significant advances have come from the analysis of the genetic variation and structure in natural populations. Many of these studies are of insects. For example the Hawaiian Drosophila , which have proliferated rapidly on the emerging islands of the archipelago, serve as an outstanding model system for examining the relations among geographic isolation, population size, sexual selection, and genetic divergence. The fact that the islands can be accurately dated in geologic time provides a unique opportunity to ascertain how the species have evolved. Founder events followed by repeated population expansions and contractions accompanied by strong sexual selection appear to have promoted the rapid divergence of isolated populations of these flies.
The causes of speciation are different in Rhagoletis , a group of economically important flies whose larvae infest the fruits of a wide range of plants. Within the past 150 years, species of these flies have formed genetically distinct host races on introduced plants, in the absence of any geographic barriers to gene flow. These races appear to be in the early stages of speciation. Detailed behavioral, ecological, biochemical, and molecular research has revealed that because mating in these flies occurs on the host fruit, genes that govern host choice directly affect mate choice.
Another approach to the study of speciation in natural populations focuses on the genetic and biological outcome of hybridization in zones of overlap either between previously geographically isolated, but closely related, populations that have reestablished contact, or in zones of transition across a sharp ecological boundary between populations adapting to different habitats. These investigations are being carried out on a wide range of animals and plants. The objective of such studies is to establish whether different mate recognition systems and reproductive isolation can evolve in zones of contact as a result of a selective process called reinforcement or develop as a by-product of genomic divergence in isolation. The increased genetic resolution recently provided by molecular techniques is contributing significantly to our understanding of how hybridization affects the speciation process.
A third approach to the study of speciation involves direct laboratory selection experiments. Such experiments suggest that considerable progress toward speciation can occur rapidly, even in the face of considerable gene flow. This experimental approach offers promise for testing some hypotheses of speciation mechanisms now being generated from studies on natural populations.
In recent years, theoretical population genetic models, using analytical and computer stimulation approaches, have been developed in an attempt to understand under what conditions species evolve in nature. These models have become increasingly more sophisticated and biologically meaningful and have yielded insights into the speciation process as well as models for exploring, in nature or in the laboratory, the conditions under which speciation can occur.
The study of speciation, one of the most important fields of research in evolutionary biology, has a direct bearing on our understanding of the origin of organismal diversity in the past, the present, and the future. It has left the descriptive, comparative phase that predominated in the past for a more empirical approach to the study of speciation mechanisms. Sufficient evidence has come from recent studies to indicate that we are on the threshold of resolving some of the most intractable problems concerning modes of speciation. The increasing interest in microbial evolution also encourages a new analysis of the species concept and species formation in prokaryotes.
The Study of Evolution of the Organization and Composition of the Genome Is Still in Its Infancy
Even though we know that the overall genetic organization of the chromosome in certain groups of bacteria is strongly conserved, the reasons are obscure. Similarly, in eukaryotes, no principles are known that govern conservation or changes in chromosome structure or organization. Genomic evolution also includes unknown contributions from various localized and dispersed highly repetitive DNA families and numerous types of transposable elements with different characteristics and evolutionary implications. In a wider sense, genomic evolution also includes that of viral genomes and the interactions with the host genomes. Recently it has become clear that certain plant genomes undergo a novel and potentially major mechanism of evolution in response to environmental stress. For example, plants under stress manifest marked phenotypic changes that are associated with heritable changes in copy number of several multigene families including ribosomal DNA sequences. New methods of manipulating and cloning large DNA molecules will be critical to the study of evolution at the level of the chromosome.
Although ambitious, the synthesis of disciplines that characterize modem evolutionary biology should be extended to embrace areas such as developmental biology, neurobiology, and behavior. Little is known about possible developmental sequences available to organisms with particular genotypes, or about new kinds of developmental pathways that are accessible by mutation from genotypes already existing. In addition, virtually nothing is known about the genetic determination of complex animal behaviors and the manner in which these behaviors feed back on the evolution of molecular and morphological traits.
- The Result Of Evolution
The Study of Adaptation Is Still a Pervasive Theme in Biology
The most dramatic result of the evolutionary process is seen in the adaptations of organisms alive today. One of Darwin's chief accomplishments in The Origin of Species was to show that the exquisite adaptations of organisms that ''so justly excite our admiration" could be explained by the purely mechanistic process of natural selection.
Important research opportunities in studies of adaptation derive from both technical and conceptual innovations during the past several decades. Some of the technical advances have been mentioned. As an example of conceptual innovation, it is now generally agreed that traits do not typically evolve for the good of the group or species as a whole, but for the direct or indirect advantages they confer on their possessor. Interdemic selection may provide an exception to this generalization, but the overall importance of interdemic selection to changing the genotypic composition of a species is unknown. The search for theories other than group selection to explain puzzling traits has led to a rich proliferation of concepts regarding, for example, selection acting not on individuals themselves but through increased fitness of their kin, and the trade-offs between fecundity and mortality in life-history strategies. However, some phenomena remain puzzling, such as that parthenogenesis does not rapidly replace sexual reproduction even though its rate of reproduction is theoretically higher.
Other conceptual advances have also enriched the study of adaptation. One is the realization that organisms often buffer themselves against changes in selection pressures. For example animals can choose species-specific microhabitats, and seeds can germinate in response to cues that signal favorable conditions. Another important concept is that of developmental constraint: the manner in which certain adaptations close off other possible paths of adaptation, thereby constraining the further evolutionary potential of the species. For example, the exoskeleton of arthropods provides attachment sites for muscles enabling rapid movement, but it also limits the maximum size of the animals.
The study of adaptation has also benefited from the integration of previously separated fields. For example, ecology and behavior are becoming increasingly integrated into evolutionary biology. By examining the genetic and phylogenetic aspects of physiological, morphological, and biochemical traits, biologists are forming bridges among evolutionary biology and physiology, development, and molecular biology.
Among numerous promising research opportunities in adaptation are studies of evolutionary and functional morphology, which increasingly includes biomechanics. Application of quantitative engineering principles combined with computer modeling has moved this field from descriptive to analytical studies. The approach enables the analysis of the specific mechanical properties of biological materials, the relation between the design of organisms and their environments, and the understanding of repeatable historical patterns in the evolution of design and the constraints placed on design by evolutionary history.
Physiological adaptations of plants and animals to factors including temperature, aridity, and osmotic stress have been abundantly analyzed by physiological ecologists, whose approach is becoming increasingly evolutionary. Indeed, some workers have begun to examine individual variation in physiological traits find to apportion the variation into genetic and nongenetic causes in attempts to determine physiological mechanisms.
Important Advances Have Been Made in Behavioral Ecology and Evolutionary Biology
The understanding of such phenomena as habitat use, food selection, social aggregation, cooperation, cannibalism, and ritualized conflict has greatly increased in the past decade. Sexual selection has become a major topic in both behavior and population genetics, and the reality of sexual selection by female choice in birds has recently been demonstrated. The next step is to test the prediction that male characteristics evolve in concert with female preference for even more exaggerated male characteristics, virtually without limit. The coevolution of male-female mate recognition characteristics may play a key role in animal speciation.
The evolution of life histories provides an active area of contact between the fields of ecology and evolution, as does the study of how interacting species adapt to each other and how such coevolution affects the structure of ecological communities. During the past decade, such studies have expanded beyond the previous emphases on competition and predation to embrace, among others, parasitism and mutualism.
The study of adaptation has been invigorated by the infusion of new concepts and theories, by an increasingly experimental and analytical approach, and by the increasing communication among fields. The incorporation of population genetic theory and phylogenetic analysis into the study of adaptation has only begun and promises to be instructive. Several hurdles must be overcome to ensure success. Tests of theories in natural populations often require considerable time—often years—before they acquire real substance; in some areas, such as physiology, techniques must be developed to automate the measurement of numerous individuals.
Although modern molecular techniques promise to contribute to an understanding of numerous unresolved questions related to the processes and history of evolution, equally important contributions will emerge from new conceptual, statistical, and technical approaches in areas such as population genetics, phylogenetic analysis, and developmental biology. Foremost among the poorly understood areas in evolution are the relations between evolutionary processes at the population level and the longer term evolutionary changes involved in the origin of species and higher taxa. A bridge between the almost separate domains of population genetics on the one hand and systematics on the other is sorely needed. Progress in building such connections may have to await advances in developmental biology and imaginative new approaches in genetics and development, but some advances in these areas hold out the promise that population and historical studies can inform each other.
For example, we may anticipate that, by the use of molecular sequences or large numbers of morphological traits or both, reasonably reliable phylogenies of groups of related species will soon be abundant. In groups that are amenable to genetic or developmental studies, the conjunction of genetic and phylogenetic or paleontological analysis offers the opportunity for studying numerous open questions. These include issues such as (1) whether rapidly evolving characteristics are more variable genetically than slowly evolving features; (2) whether genetic correlations exist between characteristics that evolve in concert across phylogenies, or whether observed phylogenetic correlations result from co-adaptation and natural selection only; and (3) whether correlations among species result from common ancestry rather than adaptation or genetic correlation. These are some of the rich fields that are available at the organismic level for the exploration of evolution and diversity.
The relation between population genetics and long-term evolution will also be strengthened as evolutionary biologists turn to developmental biology and developmental genetics. The greatest progress will come when the mechanisms of development are more fully understood. Even now we can hope for some understanding, perhaps by developmental comparisons and experiments not only between distantly related kinds of organisms such as frogs and salamanders, but also between closely related species in which hybridization or experimental transfer of genetic material may prove feasible. Among the neglected questions coming to the fore once again are, What is the mechanistic basis of the sterility of species hybrids? Are few genes responsible for hybrid sterility, or many? Why are mutations of large effect generally deleterious and what are their pleiotropic effects? Conversely, what processes are altered when gradual, polygenic changes yield a viable phenotype that may resemble the nonviable phenotype of a single mutation of large effect? What is the developmental nature of invariant or evolutionarily conservative traits, and what is their relation to the concepts of canalized phenotypes that develop in constant ways in a wide range of environments? What relations exist among the functional, phenotypic, genetic, and developmental correlations among traits?
Research in Functional Morphology and Biomechanics Has as a Major Goal the Analysis of Patterns of Diversity at the Level of Whole Organisms
Functional morphologists study mechanisms of integration of organisms, usually within both phylogenetic and evolutionary frameworks. Complex organisms are highly integrated, and the basic pattern of organization of most major taxa is conservative. This conservatism probably arises from couplings, or interlinkages among the parts of organisms that stabilize morphology. These links may be genetic (pleiotropy, genetic correlations, and so forth), developmental (inductive interactions), functional (physiological, behavioral), or structural (direct part-to-part connections). Functional morphologists examine organisms to describe such linkages. Once understood, such couplings can be used to explain why evolution is likely to proceed in certain directions rather than in others and why certain structures and functions have not evolved in the past and are unlikely to appear in the future. Thus, many functional morphologists are concerned with constraints on evolution and on opportunities that arise when such constraints are removed. Furthermore, certain evolutionary phenomena can lead to uncouplings, which may be followed by the incorporation of novelties and adaptive radiation. For example, certain salamanders lost lungs as an adaptation to live in rapidly flowing streams; the hyobranchial system was thereby uncoupled from its role as a respiratory pump and evolved into a high-speed, long-distance projectile tongue. Modem functional morphology uses a large array of methods, including high-speed video, kinematic, and x-ray cine systems for visualizing movement and behavior, electromyographic and other physiological approaches for characterizing patterns of movement, neurobiological methods such as modem staining methods for tracing neural components of integrated systems, and even quantitative genetic methods of analyzing patterns of interaction for analysis of variation within individuals.
Application of principles from the fields of materials science, engineering, cell and developmental biology, ecology, and evolutionary biology to the study of the structure and function of plants and animals has progressed rapidly and holds promise for the future. The field of biomechanics is relatively young; it differs from functional morphology in having a focus on details of structural organization and in having application from the level of cells to that of whole organisms facing the environment. The kinds of studies undertaken range from investigation of the structure of the cytoskeleton to those of the collagenous fiber wrapping of the dermis in whales and fishes and the meaning of these structures for function. Recent discoveries include the biomechanical significance of spicule arrangement in the bodies of sponges, reasons for the organization of the holdfast in giant kelps, and the means by which sea anenomes survive the battering they receive in tidal zones. Biomechanical approaches have led to new understanding of the organization and function of the notochord, of the significance of osteogenic patterns, and of the organization of muscle. Some workers span the small gap to functional morphology, while others extend their interests into surgical and other medical uses of biomechanical perspectives.
Systematics Is a Key Discipline in Evolutionary Biology
In a Chinese proverb, calling things by their proper names—systematics—is the beginning of wisdom. Modern systematics, which is basic to the study of adaptation, stresses the basic recognition and naming role, but simultaneously reaches out to all other disciplines concerned with biological diversity. Systematics comprises taxonomy—that is, surveying, recognizing, naming, describing, and making identifiable the kinds of organisms—and the development of classifications of organisms, placing them into taxa from the population to the kingdom levels. At another level of analysis it embraces the study of the relations, origins, and histories of these taxa, including the factors that led to their origin and shaped their histories.
Systematics, gradually transformed by principles and techniques from other disciplines, has the chief responsibility for analyzing diversity and putting such knowledge into a more accessible form. Cataloguing of organisms is still so incomplete that we do not even know to the nearest order of magnitude the number of species on earth. Although approximately 1.4 million species of all kinds of organisms have been formally named since Linnaeus inaugurated the binomial system of species identification in 1753, this figure grossly underestimates the diversity of life. Considering the prodigious variety of insects alone and the underrepresentation in the catalogue of many types of organisms, such as microbes, it is reasonable to guess that the absolute number of species of all groups on earth falls somewhere between 5 and 30 million.
FUNCTIONAL MORPHOLOGY AND EVOLUTIONARY ECOLOGY
Three of Africa's Great Lakes—Victoria, Tanganyika, and Malawi—are home to three remarkable species assemblages. Each lake contains 150 to 200 endemic species of the family Cichlidae, small to medium-sized sunfishlike fishes. The total of nearly 600 species represents an astonishing 3 percent—perhaps more—of the world's fish species. Why are there so many of them? Does their morphology have anything to do with their remarkable multiplicity of species?
In general, when species coexist, they are partitioning some resource—such as food or space—in such a way as to reduce competitive overlap. Such specialization is often accompanied by diversity of some physical or behavioral features of the species. Studies of the African cichlids reveal enormous diversity in two attributes: their behavior, including feeding, and the morphology of their jaws. Their feeding habits range from scraping algae from the underside of rocks to eating the scales of other fishes. One species frequently bites the eyes out of other fishes, others scrape algae from the leaves of higher plants, some eat whole fish, some eat invertebrates, and so on. Their jaws show remarkable variety in shape, size, and dentition. In addition, cichlids, like most bony fishes, have pharyngeal jaws—"throat teeth." But in cichlids, the pharyngeal jaws are more specialized and variable than in most other bony fishes.
For many years, the Great Lakes cichlids have been regarded as the showcase example of adaptive morphology associated with adaptive radiation. The unusual morphological adaptability of the jaws has been thought to have permitted the remarkable adaptive radiation observed. Certain morphological characteristics of their pharyngeal jaws have allowed those jaws to become adapted to some of the functions usually performed by the mouth jaws. This has freed the mouth jaws to become diversified to perform unique food-gathering functions, almost like a hand; the mouth jaws also have characteristics that seem to allow greater diversification of function than those of most other bony fishes.
The above interpretation is the more plausible because radiation has occurred three separate times in the three lakes. There is even a natural control: Several other families of fishes that lack the cichlids' jaw adaptations inhabit the same lakes but have not radiated similarly.
But recently, puzzling questions have been raised. For example, the cichlid family is represented in the African lakes by two subfamilies, both of which have the specialized pharyngeal and mouth jaws discussed above, specializations presumed to have allowed the great speciation observed. But the explosive speciation has occurred primarily in one subfamily, the Haplochromines. The tilapine subfamily has relatively few species. Why? Another question concerns the characoid fishes of Amazonia, a group of fishes containing the piranhas. They, like the African cichlids, have enormous numbers of species, but they lack the specializations of the cichlids' jaws.
Finally, it has recently become clear that the behavioral and morphological diversity of the African cichlids is strongly influenced by environmental factors. This means that differences observed in nature might not be entirely—or even mostly—genetically based.
The African cichlids remain, as they have long been considered, of enormous evolutionary interest. But, rather than being a textbook example of any one particular phenomenon, they seem to represent a natural laboratory for studying evolution, ecology, and morphology. And that study is still in its exciting early stages.
Proper species classification is important because a species is not like a molecule in a cloud of molecules, but is rather a unique population of organisms; the terminus of a lineage that split off from the most closely related species thousands or even millions of years ago. Species have been shaped into their present forms by mutations and natural selection, during which certain genetic combinations survived and reproduced differentially out of an almost inconceivably larger total. No two species, no matter how closely related, are any more interchangeable than are two Mozart sonatas. Each species of organism is incredibly rich in genetic information. The genetic information in the constituent bases that make up the DNA in a single mouse cell, if translated into ordinary letters of printed text, would nearly fill all 15 editions of the Encyclopedia Britannica published since 1768.
Since other evolutionary disciplines, including ecology, biogeography, and behavioral biology, depend on systematics, an entire hierarchy of important problems must be addressed. Two stand out in the sense that progress toward their solutions is needed to put the other disciplines on a permanently sound basis. The first problem is to define the magnitude and causes of biological diversity, and the second is to determine the most reliable measures of homology and their implications for phylogenetic relationships.
In defining the magnitude and causes of biological diversity, systematics will undoubtedly fall short of obtaining a complete catalog of life on earth, but a determined effort would pay many dividends. A greater understanding of biological diversity promises to resolve some of the conflicts in current theory and at the same time to open productive new areas of research. In addition, the answers will influence a variety of related disciplines, affect our view of the place of humans in the order of things, and open opportunities for the development of new knowledge of social importance. For example, control of mosquito-borne diseases such as malaria has profited from the ability to define as separate species mosquitoes that are morphologically almost identical but that differ in behavior and in their ability to transmit the diseases.
Systematics is also a discipline with a time limit because much diversity is being lost through extinction caused by the accelerating destruction of natural habitats. This is especially true in the tropical moist forests, where more than half of the world's species are thought to exist. Although extinction rates are difficult to estimate, in part owing to inadequate systematics, current rates of extinction seem to approach or exceed 1,000 times the average rate in past geological time. Tragically, and perhaps ominously for human welfare, most of the tropical forests, and with them many thousands of species of plants and animals, seem destined to disappear during the next 30 years. It is not too much to say that humanity is locked into a race in which systematics must play a crucial role.
From a practical standpoint, plants provide many critical medicines and pharmaceuticals, many species contain genes for disease resistance and other desirable traits that can potentially improve agricultural varieties, and many could potentially be developed into important crops themselves. For example, the taxonomy of plants has stimulated and in turn been invigorated by the discovery of more than 10,000 secondary compounds scattered among a vast array of species. These substances (alkaloids, terpenes, phenolics, cyanogens, and glucosinolates) are equally crucial to the understanding of plant evolution and to the improvement of human welfare. Thus, the study of biological diversity and the desire for its preservation are not based on esthetic principles alone.
Systematics Also Includes Studies of the Interrelationships Among Organisms
Studies on phylogenetic relationships among organisms aid in the development and evaluation of theories about evolutionary processes. Models of the origin of species have been stimulated as well as guided by the development of the species concept in systematics. Phylogenetic information is important in many areas of evolutionary biology. For example, in biogeography, the occurrence of flightless ratite birds (ostrich, rhea, emu, and others) in Africa, South America, Australia, and New Zealand is apparently inconsistent with morphological and molecular data indicating that the birds share a common ancestry. The paradox is resolved by the knowledge that the birds all diverged from a common stock that inhabited the great southern continent of Gondwanaland before it split and became dispersed through continental drift. Phylogenetic information is the basis of the comparative method for the study of adaptation. A positive adaptive value for a particular characteristic is suggested when two or more distantly related organisms have undergone parallel evolution in that characteristic, for example flower shape or color. Phylogenetic analysis is also necessary for understanding the sequence in which characteristics have undergone evolutionary transformation and for estimating rates of evolutionary change, be it morphological or molecular.
One of the chief tasks of systematics is the elucidation of phylogenetic, or genealogical, relationships among organisms. Inference of genealogy is a desirable goal both for fossilized forms and for living organisms whose ancestry is poorly documented in the fossil record. The reconstruction of phylogenetic history is often of great interest in itself, for example in determining the ancestral relationships among humans and other higher primates. But the reconstruction of phylogenetic trees has numerous other uses as well. Indeed, phylogenetic data are the source of almost everything we know about the patterns of evolutionary change over the course of millions of years, including convergent evolution, parallel evolution, adaptive radiation, and mosaic evolution. Phylogenetic studies have been essential to understanding how species have arrived at their present geographical distributions and to interpreting processes and rates of change at the level of the DNA.
Only in the past 20 years have the logical and evidential criteria for establishing phylogenetic relationships been articulated. Through these sometimes controversial developments, systematics has become a highly sophisticated, rigorous science in which mathematics, statistics, and molecular biology play leading roles. Modern systematics differs greatly from what it was even 10 years ago and poses extremely complex questions.
An important step in this revolution was the development of methods of classification that allowed treelike diagrams expressing the similarity among organisms to be derived by objective criteria through the use of appropriate mathematical expressions evaluated by computer algorithms. These kinds of clustering procedures first developed for biological classification have since been used in many other applications, for example, in linguistics.
The treelike diagrams derived from clustering procedures do not necessarily reflect the genealogical relationships in phylogenetic trees unless the similarity of two species is directly proportional to how recently they diverged from their common ancestor. Proportionality does not exist when many characteristics undergo convergent evolution or when different evolutionary lineages evolve at different rates. However, methods have also been developed that aim to infer the correct phylogenetic relationships among species, although these methods are sometimes difficult to apply in practice because of uncertainties and ambiguities in the data. An important area of current research is the development of statistical techniques to evaluate the degree of uncertainty in estimates of phylogenetic relationship. Just as estimates of numerical quantities should be accompanied by confidence intervals giving the precision of the estimates, inferred patterns of phylogenetic relationship must be accompanied by some kind of measure of their reliability.
Traditionally relying on the data most readily available, usually the morphological characteristics of preserved museum specimens, modern systematics also includes other sources of data, such as ecology, behavior, genetics, and biochemistry. The power of systematics has recently been augmented by data from molecular biology. Electrophoretically distinguishable proteins are now routinely used to distinguish species and to estimate phylogenetic relationships among closely related species, and restriction enzyme digests of DNA sequences such as mitochondrial DNA provide numerous systematic characters.
- Evolutionary History
The Fossil Record Makes Special Contributions to Evolutionary Biology and to Knowledge of Present-Day Diversity
Although questions of both process and result are central in evolution and diversity, the history of evolution has only one source of primary direct evidence, one court of last resort, which is the fossil record. Studies in paleobiology therefore directly affect all aspects of evolution and diversity.
The fossil record provides the vital time dimension for the understanding of biological diversity and the history of life. The current data base of paleobiology consists of records of some 250,000 extinct species of plants, animals, and microorganisms occurring in deposits spanning more than 3.5 billion years of earth history. Although the record comprises only a small fraction of all the fossil taxa that ever lived, systematic collections in museums and universities contain tens of millions of documented specimens, in many cases with good representation of individual species in space and time.
With respect to the history of diversity, the fossil record can be analyzed to determine whether diversity is higher now than in the geological past, whether the evolution of diversity might be expected to reach a steady-state level, and whether community structure has changed over geological time.
Paleobiology is unique in being the only source of data about certain evolutionary processes and events. For example, although the observational and experimental work of most biologists is necessarily limited to processes and phenomena that are relatively rapid or common, paleobiologists capitalizing on the depth of the geological record have access to much rarer events.
The geological record also documents a unique and lengthy natural experiment in adaptation. Many biological innovations originated, flourished, and died out long before the modem biota emerged. Studies in paleobiology can shed light on when these lost adaptations originated and whether they were better solutions to functional problems than are found among living organisms today.
Adaptive radiations—bursts of speciation in which the number of species in a biological group or adaptive zone increases exponentially during a relatively short time, with accompanying expansion in the diversity of structure and function—is well documented in the fossil record, but it is not clear whether these grand adaptive radiations are analogous to the smaller-scale bursts of speciation observed, for example, among Hawaiian drosophilids and African cichlid fish.
THE RISE OF THE ANTS
In 1967, Harvard University received the first known specimens of fossil ants of Mesozoic age, two beautifully preserved specimens in the clear orange amber from a redwood tree that grew 80 million years ago in New Jersey. These specimens were something of a breakthrough in the study of insect evolution. Until then the oldest known fossils were about 30 to 40 million years old (from the Oligocene epoch) and quite modern in aspect. The main features of ant evolution had already been fleshed out. The only phylogenetic tree that could be drawn from such evidence was the canopy, with the trunk and roots cut off. The Mesozoic ants provided what appeared to be a piece of the trunk.
Soon afterward, Soviet paleontologists began to describe a long series of other antlike fossils, also about 80 million years old, giving a separate scientific name to almost every specimen. When all these bits and pieces were fitted together and the New Jersey fossils added in 1986, a remarkable picture emerged: The specimens fell into three classes, representing the worker caste, the queen caste, and the male of the most primitive ants. The workers lacked wings and had proportionately small abdomens, the hallmarks of a sterile caste. These fossils made it possible to conclude that social life had been established in the ants by 80 million years ago, a startling conclusion in view of the earlier lack of such ancient fossils.
A close examination of the American and Soviet fossils showed them to be similar to what had been expected for ancestral ants. Their anatomy was a mosaic of traits, some typical of nonsocial wasps and some more modern—but still typical of generalized ants. They provided the first clue concerning the group of wasps from which ants arose.
The Harvard collection recently obtained the first ant fossils of mid-Eocene age, from Arkansas this time. Chinese and Soviet paleontologists were close behind, discovering Eocene specimens from Manchuria and Sakhalin, respectively. These ants are thought to be 50 to 60 million years old, and most of them are very different from the Mesozoic fossils. They are diverse, representing both modern taxonomic groups and (in one case) a stock not too distant from the Mesozoic ants. It thus seems that the ants, like the mammals, crossed a threshold around the end of the Mesozoic era. For some reason not yet understood, they expanded into a richly various, world-dominant group.
Entomologists and paleontologists continue to search avidly for fossils from Mesozoic and early Cenozoic deposits. The questions we hope to answer include when, where, and from which wasplike insects the ants arose; exactly when they radiated into their modern aspect; the directions they took when spreading around the world; and, not least, what traits contributed to their spectacular success.
Extinction Has Been the Fate of Almost All Species That Have Ever Lived
Extinction, as a biological process, is difficult to study in modern environments. Although the background rate of extinction is low—estimated as about one global extinction per million species per year—extinction is not only frequent on a geological time scale but has been responsible for many complete turnovers in the biological composition of the earth. A proper understanding of the evolutionary process is impossible without knowledge of rates of extinction, quite apart from the importance of such knowledge in evaluating the magnitude of the increase in rates of extinction resulting from human activities in modern times.
Understanding the environmental causes and evolutionary implications of the occasional, brief periods of mass extinction in earth history is a key problem in paleobiology. The most severe mass extinction occurred 250 million years ago and eliminated between 75 and 95 percent of the species then alive. In short, the global biota had a close call with total annihilation. Somewhat less severe mass extinctions are scattered throughout the fossil record. Recent work on the likelihood that some mass extinctions were caused by meteorite impact shows promise of establishing strong connections between biological evolution and the cosmic environment. When combined with the more speculative possibility that impact-induced extinctions are regularly periodic, this hypothesis opens the possibility for major shifts in the way the evolution of the global biota is interpreted.
Within the past 2 million years of the fossil record, constituting the Pleistocene epoch, are special opportunities for studies of biological diversity. During this period, the biota was essentially modem but subjected to the effects of well-documented major changes in climate and geography that set the stage for the present distribution of plant and animal species. Modern tropical rain forests, to pick just one example, can be understood only by knowing the historical underpinnings that led to their present distribution and composition. This understanding is critical in developing a strategy for dealing with the effects of human activities, especially in the moist tropics.
Palcobiologists Have Made Major Progress in the Past Two Decades
Research results have been astounding, at the other end of the time scale, in deciphering the oldest records of life on earth. Not only did life begin far earlier than biologists had previously envisaged, but, perhaps even more surprising, the earth's biota was composed solely of bacteria over such an extended period. These fossil discoveries have recast concepts of evolution and diversity and have reemphasized the fact that, when viewed over the long sweep of geological time, a significant part of evolutionary progress has resulted from changes in the intracellular biochemistry of bacteria.
Paleobiology includes several research areas that have special promise of making significant contributions to evolutionary biology and to other fields of the natural sciences. Among these are the origin of life itself, including not only when life began, by what processes and in what types of environments, but also whether life might exist elsewhere in our solar system or in the universe. Such issues are ripe for exploration during the coming decade because recent progress in studies of ancient Precambrian fossils has extended the known record of life on earth to more than 3.5 billion years. Studies of even more ancient deposits, coupled with laboratory studies of chemical reactions that can occur in a lifeless environment and biochemical studies of existing microbial organisms, promise to provide new evidence of the beginnings of life and of the environment in which life originated.
Organisms Alive Today Are Well Adapted to the Vagaries of Their Present-Day Environment
Environmental conditions such as atmospheric composition, day-night light regime, and temperature conditions have changed markedly over the course of geological time. Until about 1.7 billion years ago, well after the origin of living systems, the atmosphere contained too little oxygen to sustain obligately air-breathing forms of life. Day length has progressively lengthened as the distance between the earth and moon has gradually increased, and there is good evidence that the earth's average surface temperature has changed markedly. Each evolving species became adapted to the environment in which it originated, and as the environment changed, life evolved and built on foundations that had become established under earlier regimes. Therefore, recorded in the genetics, biochemistry, cellular structure, and gross anatomy of living organisms may be a coded history of their evolution. For example, analyses of growth bands in fossil corals and mollusks have made it possible to track the changes in day length caused by tidal friction. Even more spectacular has been the recent recognition of Milankovich cycles of climatic change over the past 700,000 years, which almost certainly were responsible for the pulses of continental glaciation during the Pleistocene epoch.
Deep-sea drilling during the past two decades has provided continuous sections in which important population-level analyses of evolutionary changes are feasible. This increased resolution in the fossil record introduces a time scale comparable to that of microevolutionary change in population genetics, and it opens a more complete synthesis of these two disciplines. The oceanic fossil record is excellent for the last 160 million years, and the deep-sea cores provide a rich source of information on the evolution of single-species lineages. Statistical analyses have already documented important patterns of morphological change and the not-uncommon lack of such change known as stasis. But the surface of this field has only been scratched by the investigations carried out thus far, and we have much more to learn about the tempo and mode of evolution.
Much also remains to be learned regarding the timing and nature of major evolutionary events. Some of them, such as the origin of invertebrates, vertebrates, flowering plants, angiosperms, and humans, have been recognized as important research problems since the mid-nineteenth century. Other events, such as the advent of photosynthesis, oxygen-dependent respiration, the anaerobic-aerobic global ecosystem, nucleated cells, and eukaryotic sexual reproduction, have been addressed only recently with the upsurge of interest in the Precambrian fossil record. Future studies will promote a better understanding of the timing and context of major evolutionary events in the history of life on earth.
- Current Status Of Research
Contemporary Research in Evolution and Diversity Features Several Exciting Growing Points
A particularly promising field spanning the synthesis of molecular biology and evolutionary biology is expected to reveal new evolutionary principles even as it resolves some longstanding issues. Important as this new synthesis is, it must be emphasized that not everything in evolution and diversity can be reduced to molecular biology.
Many central issues of evolution at the organismic level require different kinds of approaches. These include the study of the evolution of complex multifactorial traits within populations and the evolutionary role of selection among populations. Innovative approaches to uniting physiology, behavior, and development should also be encouraged.
Those who set research priorities in evolution and diversity must also recognize the continuing importance of cataloguing the diversity of life on earth and understanding its origins through speciation and its disappearance through extinction. Apart from the scientific value of such research are the many potential practical applications of the findings in medicine, agriculture, and biotechnology. Groups of organisms that are already relatively well known, such as vertebrates, plants, and butterflies, are important to study because of the light further information about them would shed on overall biogeographic problems. In addition, economically important groups of organisms, such as legumes and mosquitoes, should be emphasized in choosing priorities for study. Areas of vegetation that are already decimated and those that are being destroyed rapidly but that contain large numbers of endemic species should also receive special emphasis. Concerted efforts to survey more or less completely the biota of selected places, especially in the disappearing forests of the tropics, would be much more rewarding than miscellaneous sampling of poorly known groups over wide areas. Greater attention should also be given to groups that are especially tractable for the solution of basic problems in ecology, population biology, and evolution. To accomplish this, additional systematic biologists must be trained and employed, since the current world supply is much too limited to attack the millions of species of unknown or poorly known organisms profitably.
Paleobiology also presents significant new opportunities for breakthroughs in understanding the history of life on earth, including its earliest history in the Precambrian, its diversification and geographical distribution, and its extinction through, in some cases, global processes.
Collections and Special Facilities
Museums are one logical place to concentrate the effort to encompass diversity.
These institutions are already the repositories of vast numbers of priceless specimens, often representing species that are endangered or recently extinct. Yet most of the collections are fallow, and the halls of some of the leading research museums are largely empty of qualified researchers. The same is true of zoos and botanical gardens, which are in effect museums filled with living specimens. One of the premier tropical botanical gardens in the continental United States has purchased no major items of research equipment in 20 years. Although it averages only one postdoctoral fellow per year, it could easily accommodate six.
An additional need exists for regional or international centers for the storage and analysis of fossil pollen and other microfossils, which are vital in the reconstruction of evolutionary histories and past environmental change. For example, we are only now becoming aware of the considerable extinction of species that has been caused by human disturbances, especially on islands, lakes, and other geographically restricted habitats. One of the most promising domains of research is the detailed analysis of this impoverishment during the past several thousand years, with an emphasis on the factors that make certain species more vulnerable than others.
The future of systematics and its contribution to evolutionary studies depend on collaboration among workers in different fields, funding of interdisciplinary studies, and mutual education. Museums, the traditional home of systematics, will find it necessary to expand their facilities and personnel to encompass statistical, molecular, and experimental approaches. The traditionally modest sums granted to systematists will not support molecular investigations, and it will be useful to set up facilities for molecular systematics that can be used by multiple workers. Above all, university biology departments, in their staffing and curricular decisions, must take into account the growing impact of the new systematics on the study of evolution and its implications throughout biology, from molecular biology to ecology.
Museums are also vital to the continued health of research on the fossil record by maintaining and developing systematic collections. These Collections are the lifeblood of research progress. Research questions change continually, and it is important that museum collections remain an effective source of empirical data and that the data be actively studied and described by competent specialists.
The paleontological collections of the United States are in reasonably good shape, thanks to many years of financial support from the Biological Research Resources program of the National Science Foundation. Continued support is critical to sustain active research programs of relevance to broader problems of evolutionary biology. Museum collections are becoming especially critical in some areas because of the phasing out of support for collections by many major research universities.
Collection and Conservation of Germplasm Is Crucial For Improved Agricultural Production
Human activities associated with modern civilizations are causing a loss of diversity at all levels of biological organization. Once lost, this store of genetic diversity can never be recovered. A case in point is the loss of genetic diversity associated with the primitive land races (plants that are adapted to a region in which they evolved) and wild relatives of our crop plants. These genetic resources have repeatedly provided genes for disease resistance when agriculture has been challenged by serious disease epidemics, and these resources constitute an important source of novel phenotypes in conventional plant improvement. They must be found and conserved for our common good.
Modem agriculture is characterized by extensive plantings of genetically uniform monocultures. For example, genetically uniform hybrid corn is widely grown in the United States. Genetically uniform populations have the advantages of high yields, uniform size, and uniform dates of maturity, and these features have played a major role in the great increase in productivity of agriculture in the United States during the past 50 years. Uniformity of size and maturity are also required by highly mechanized agricultural practices.
On the downside, genetically uniform crops are vulnerable to large losses from pest or disease outbreaks because monocultures may lack the genetic variability for resistance to the pathogens. In 1970, a fungal pathogen raced through the U.S. corn crop in the corn leaf-blight epidemic. It was quickly discovered that susceptibility to the fungal pathogen was associated with a particular mitochondrial genotype that had been widely incorporated into breeding stocks. Luckily, other mitochondrial genotypes conferred resistance, and the resistant genotypes were introduced into commercial lines of corn. By 1971 corn varieties resistant to the leaf blight had largely replaced the susceptible type in agricultural production, and the corn crop was protected. The resistant types were available because an international effort had been made to conserve plant genetic resources for just such contingencies.
A second major disadvantage associated with the wide, and in some cases nearly global, adoption of monocultures is that these plantings supplant and drive to extinction the wild relatives and primitive cultivated forms of crop plants, which provide a source of genetic variants for future breeding efforts. Genetic conservation is faced with two problems—how to save and maintain useful plant germplasm and how to evaluate plant gene pools in order to preserve as wide a sample of potentially useful genetic variants as possible. The problem of evaluation is particularly difficult because we have no way of predicting which kinds of novel genetic variants the future may require. At present, the best that can be done is to evaluate the plants of interest for a wide range of genetic traits and select a sample for conservation that includes as much diversity as possible. Little is known about the adequacy and scope of contemporary germplasm collections. Genetic screening procedures and statistical sampling plans need to be developed for this task.
Finally, what little effort is expended to protect plants is almost entirely devoted to crop plants and their wild relatives—about 150 species out of the more than 260,000 kinds of plants known. Botanists estimate that tens of thousands of kinds of plants could probably be developed into useful crops-not only for food but also as sources of medicines, oils, waxes, and other chemicals of industrial importance-if we would carry out the appropriate investigations, identify them, and develop them according to their cultural requirements. Virtually no effort is being expended in such investigations; yet fully a quarter of all plant species, along with a similar proportion of animals and microorganisms, are in danger of extinction. Even if the techniques of genetic engineering are fully applied to the development of new kinds of crops, there will need to be a source of appropriate genes; the plants that we are passively allowing to become extinct could well provide such genes, and we should find and conserve them while they still exist.
- Cite this Page National Research Council (US) Committee on Research Opportunities in Biology. Opportunities in Biology. Washington (DC): National Academies Press (US); 1989. 8, Evolution and Diversity.
- PDF version of this title (4.1M)
In this Page
Recent activity.
- Evolution and Diversity - Opportunities in Biology Evolution and Diversity - Opportunities in Biology
Your browsing activity is empty.
Activity recording is turned off.
Turn recording back on
Connect with NLM
National Library of Medicine 8600 Rockville Pike Bethesda, MD 20894
Web Policies FOIA HHS Vulnerability Disclosure
Help Accessibility Careers
133 Biodiversity Topics & Examples
🔝 top-10 biodiversity topics for presentation, 🏆 best biodiversity project topics, 💡 most interesting biodiversity assignment topics, 📌 simple & easy biodiversity related topics, 👍 good biodiversity title ideas, ❓ biodiversity research topics.
- Biodiversity loss.
- Global biodiversity conservation.
- The Amazon rainforest.
- Animal ecology research.
- Sub Saharan Africa.
- Marine biodiversity.
- Threats to ecosystems.
- Plant ecology.
- Importance of environmental conservation.
- Evolution of animal species.
- Biodiversity Benefits for Ecology This variation of species in the ecosystem is a very important concept and factor that indeed is the basis for sustaining life on our planet. Moreover, the most important supporter of life, which is soil […]
- Habitat Destruction and Biodiversity Extinctions The instance of extinction is by and large regarded as the demise of the very last character of the genus. Habitat obliteration has played a major part in wiping out of species, and it is […]
- Biodiversity Conservation: Tropical Rainforest The forest is not a threat to many species and that, therefore, helps in showing that conserving this forest will be of great benefit to many species. The disadvantage of conserving the Mangrove Forest is […]
- Biodiversity Hotspots: The Philippines The International Conservation has classified the Philippines as one of the biodiversity hotspots in the world. Additionally, the country is said to be one of the areas that are endangered in the world.
- Marine Biodiversity Conservation and Impure Public Goods The fact that the issue concerning the global marine biodiversity and the effects that impure public goods may possibly have on these rates can lead to the development of a range of externalities that should […]
- Climate Change’s Negative Impact on Biodiversity This essay’s primary objective is to trace and evaluate the impact of climate change on biological diversity through the lens of transformations in the marine and forest ecosystems and evaluation of the agricultural sector both […]
- The Importance of Biodiversity in Ecosystem The most urgent problem right now is to maintain the level of biodiversity in this world but it has to begin with a more in-depth understanding of how different species of flora and fauna can […]
- Aspects, Importance and Issues of Biodiversity Genetic diversity is a term used to refer to the dissimilitude of organisms of the same species. Species diversity is used to refer to dissimilitude of organisms in a given region.
- Introduced Species and Biodiversity Rhymer and Simberloff explain that the seriousness of the phenomenon may not be very evident from direct observation of the morphological traits of the species.
- Loss of Biodiversity and Extinctions It is estimated that the number of species that have become extinct is greater than the number of species that are currently found on earth.
- Biology Lab Report: Biodiversity Study of Lichens As a consequence of these results, the variety of foods found in forest flora that include lichens may be linked to varying optimum conditions for establishment and development.
- How Biodiversity Is Threatened by Human Activity Most of the marine biodiversity is found in the tropics, especially coral reefs that support the growth of organisms. Overexploitation in the oceans is caused by overfishing and fishing practices that cause destruction of biodiversity.
- Essentials of Biodiversity At the same time, the knowledge and a more informed understanding of the whole concept of biodiversity gives us the power to intervene in the event that we are faced by the loss of biodiversity, […]
- Conserving Biodiversity: The Loggerhead Turtle The loggerhead sea turtle is the species of oceanic turtle which is spread all over the world and belongs to the Cheloniidae family.
- Biodiversity and Food Production This paper will analyze the importance of biodiversity in food production and the implications for human existence. Edible organisms are few as compared to the total number of organisms in the ecosystem.
- Biodiversity and Dynamics of Mountainous Area Near the House It should be emphasized that the term ecosystem used in this paper is considered a natural community characterized by a constant cycle of energy and resources, the presence of consumers, producers, and decomposers, as well […]
- Biodiversity: Aspects Within the Sphere of Biology Finally, living objects consist of cells, which are the basic units of their function and structure. The viruses’ structure depends on which nucleic acid is included, which denotes that there are DNA and RNA viruses.
- Coral Reef and Biodiversity in Ecosystems Coral reefs are formed only in the tropical zone of the ocean; the temperature limits their life – are from +18 to +29oS, and at the slightest deviation from the boundaries of the coral die.
- Biodiversity and the Health of Ecosystems Various opinions are revealed concerning biodiversity, including the human impact, reversal of biodiversity loss, the impact of overpopulation, the future of biodiversity, and the rate of extinction.
- Wild Crops and Biodiversity Threats However, out of millions of existing types of wild crop cultures, the vast majority have been abandoned and eradicated, as the agricultural companies placed major emphasis on the breeding of domesticated cultures that are easy […]
- Biodiversity, Interdependency: Threatened and Endhangered Species In the above table, humans rely on bees to facilitate pollination among food crops and use their honey as food. Concurrently, lichens break down rocks to provide nutrient-rich soil in the relationship.
- Invasive Processes’ Impact on Ecosystem’s Biodiversity If the invasive ones prove to be more adaptive, this will bring about the oppression of the native species and radical changes in the ecosystem.
- National Biodiversity Strategy By this decision, the UN seeks to draw the attention of the world community and the leaders of all countries to the protection and rational use of natural resources.
- Rewilding Our Cities: Beauty, Biodiversity and the Biophilic Cities Movement What is the source of your news item? The Guardian.
- Restoring the Everglades Wetlands: Biodiversity The Act lays out the functions and roles of the Department of Environmental Protection and the South Florida Water Management District in restoration of the Everglades.
- Biodiversity: Importance and Benefits This is due to the fact that man is evolving from the tendency of valuing long term benefits to a tendency of valuing short terms benefits.
- A Benchmarking Biodiversity Survey of the Inter-Tidal Zone at Goat Island Bay, Leigh Marine Laboratory Within each quadrant, the common species were counted or, in the case of seaweed and moss, proliferation estimated as a percentage of the quadrant occupied.
- Biodiversity: Population Versus Ecosystem Diversity by David Tilman How is the variability of the plant species year to year in the community biomass? What is the rate of the plant productivity in the ecosystem?
- Biodiversity Hotspots and Environmental Ethics The magnitude of the problem of losing biodiversity hotspots is too great, to the extend of extinction of various species from the face of the earth.
- Natural Selection and Biodiversity These are featured by the ways in which the inhabiting organisms adapt to them and it is the existence of these organisms on which the ecosystems depend and therefore it is evident that this diversity […]
- Loss of Biodiversity in the Amazon Ecosystem The growth of the human population and the expansion of global economies have contributed to the significant loss of biodiversity despite the initial belief that the increase of resources can halt the adverse consequences of […]
- Biodiversity: American Museum of Natural History While staying at the museum, I took a chance to visit the Milstein Family Hall of Ocean Life and the Hall of Reptiles and Amphibians.
- Urban Plants’ Role in Insects’ Biodiversity The plants provide food, shelter and promote the defensive mechanisms of the insects. The observation was also an instrumental method that was used to assess the behavior and the existence of insects in relation to […]
- Biodiversity Markets and Bolsa Floresta Program Environmentalists and scholars of the time led by Lord Monboddo put forward the significance of nature conservation which was followed by implementation of conservation policies in the British Indian forests.
- Brazilian Amazonia: Biodiversity and Deforestation Secondly, the mayor persuaded the people to stop deforestation to save the Amazon. Additionally, deforestation leads to displacement of indigenous people living in the Amazonia.
- Defining and Measuring Biodiversity Biodiversity is measured in terms of attributes that explore the quality of nature; richness and evenness of the living organisms within an ecological niche.
- Biodiversity, Its Importance and Benefits Apart from that, the paper is going to speculate on the most and least diverse species in the local area. The biodiversity can be measured in terms of the number of different species in the […]
- Biodiversity, Its Evolutionary and Genetic Reasons The occurrence of natural selection is hinged on the hypothesis that offspring inherit their characteristics from their parents in the form of genes and that members of any particular population must have some inconsiderable disparity […]
- Biodiversity Hotspots: Evaluation and Analysis The region also boasts with the endangered freshwater turtle species, which are under a threat of extinction due to over-harvesting and destroyed habitat.
- Natural Sciences: Biodiversity and Human Civilisation The author in conjunction with a team of other researchers used a modelling study to illustrate the fact approximately 2 percent of global energy is currently being deployed in the generation of wind and solar […]
- How Human Health Depends on Biodiversity The disturbance of the ecosystem has some effects on the dynamics of vectors and infectious diseases. Change of climate is a contributing factor in the emergence of new species and infectious diseases.
- Biodiversity and Business Risk In conclusion, biodiversity risk affects businesses since the loss of biodiversity leads to: coastal flooding, desertification and food insecurity, all of which have impacts on business organizations.
- Measurement of Biodiversity It is the “sum total of all biotic variation from the level of genes to ecosystems” according to Andy Purvus and Andy Hector in their article entitled “Getting the Measure of Diversity” which appeared in […]
- Ecosystems: Biodiversity and Habitat Loss The review of the topic shows that the relationship between urban developmental patterns and the dynamics of ecosystem are concepts that are still not clearly understood in the scholarly world as well as in general.
- When Human Diet Costs Too Much: Biodiversity as the Ultimate Answer to the Global Problems Because of the unreasonable use of the natural resources, environmental pollution and inadequate protection, people have led a number of species to extinction; moreover, due to the increasing rates of consumerist approach towards the food […]
- The Impact of Burmese Pythons on Florida’s Native Biodiversity Scientists from the South Florida Natural Resource Center, the Smithsonian institute and the University of Florida have undertaken studies to assess the predation behavior of the Burmese pythons on birds in the area.
- Threat to Biodiversity Is Just as Important as Climate Change This paper shall articulate the truth of this statement by demonstrating that threats to biodiversity pose significant threat to the sustainability of human life on earth and are therefore the protection of biodiversity is as […]
- Cold Water Coral Ecosystems and Their Biodiversity: A Review of Their Economic and Social Value
- Benchmarking DNA Metabarcoding for Biodiversity-Based Monitoring and Assessment
- Prospects for Integrating Disturbances, Biodiversity and Ecosystem Functioning Using Microbial Systems
- Enterprising Nature: Economics, Markets, and Finance in Global Biodiversity Politics
- Institutional Economics and the Behaviour of Conservation Organizations: Implications for Biodiversity Conservation
- Fisheries, Fish Pollution and Biodiversity: Choice Experiments With Fishermen, Traders and Consumers
- Last Stand: Protected Areas and the Defense of Tropical Biodiversity
- Hardwiring Green: How Banks Account For Biodiversity Risks and Opportunities
- Governance Criteria for Effective Transboundary Biodiversity Conservation
- Marine Important Bird and Biodiversity Areas for Penguins in Antarctica: Targets for Conservation Action
- Ecological and Economic Assessment of Forests Biodiversity: Formation of Theoretical and Methodological Instruments
- Environment and Biodiversity Impacts of Organic and Conventional Agriculture
- Food From the Water: How the Fish Production Revolution Affects Aquatic Biodiversity and Food Security
- Biodiversity and World Food Security: Nourishing the Planet and Its People
- Climate Change and Energy Economics: Key Indicators and Approaches to Measuring Biodiversity
- Conflicts Between Biodiversity and Carbon Sequestration Programs: Economic and Legal Implications
- Models for Sample Selection Bias in Contingent Valuation: Application to Forest Biodiversity
- Optimal Land Conversion and Growth With Uncertain Biodiversity Costs
- Internalizing Global Externalities From Biodiversity: Protected Areas and Multilateral Mechanisms of Transfer
- Combining Internal and External Motivations in Multi-Actor Governance Arrangements for Biodiversity and Ecosystem Services
- Balancing State and Volunteer Investment in Biodiversity Monitoring for the Implementation of CBD Indicators
- Differences and Similarities Between Ecological and Economic Models for Biodiversity Conservation
- Globalization and the Connection of Remote Communities: Household Effects and Their Biodiversity Implications
- Shaded Coffee and Cocoa – Double Dividend for Biodiversity and Small-Scale Farmers
- Spatial Priorities for Marine Biodiversity Conservation in the Coral Triangle
- One World, One Experiment: Addressing the Biodiversity and Economics Conflict
- Alternative Targets and Economic Efficiency of Selecting Protected Areas for Biodiversity Conservation in Boreal Forest
- Analysing Multi Level Water and Biodiversity Governance in Their Context
- Agricultural Biotechnology: Productivity, Biodiversity, and Intellectual Property Rights
- Renewable Energy and Biodiversity: Implications for Transitioning to a Green Economy
- Agricultural Biodiversity and Ecosystem Services of Major Farming Systems
- Integrated Land Use Modelling of Agri-Environmental Measures to Maintain Biodiversity at Landscape Level
- Changing Business Perceptions Regarding Biodiversity: From Impact Mitigation Towards New Strategies and Practices
- Forest Biodiversity and Timber Extraction: An Analysis of the Interaction of Market and Non-market Mechanisms
- Poverty and Biodiversity: Measuring the Overlap of Human Poverty and the Biodiversity Hotspots
- Protecting Agro-Biodiversity by Promoting Rural Livelihoods
- Maintaining Biodiversity and Environmental Sustainability
- Landscape, Legal, and Biodiversity Threats That Windows Pose to Birds: A Review of an Important Conservation Issue
- Variable Mating Behaviors and the Maintenance of Tropical Biodiversity
- Species Preservation and Biodiversity Value: A Real Options Approach
- What Is Being Done to Preserve Biodiversity and Its Hotspots?
- How Are Argentina and Chile Facing Shared Biodiversity Loss?
- Are Diverse Ecosystems More Valuable?
- How Can Biodiversity Loss Be Prevented?
- Can Payments for Watershed Services Help Save Biodiversity?
- How Can Business Reduce Impacts on the World’s Biodiversity?
- Are National Biodiversity Strategies Appropriate for Building Responsibilities for Mainstreaming Biodiversity Across Policy Sectors?
- How Does Agriculture Effect Biodiversity?
- Are There Income Effects on Global Willingness to Pay For Biodiversity Conservation?
- How Does the Economic Risk Aversion Affect Biodiversity?
- What Are the Threats of Biodiversity?
- How Has the Increased Usage of Synthetic Pesticides Impacted Biodiversity?
- What Does Drive Biodiversity Conservation Effort in the Developing World?
- How Does the Plantation Affect Biodiversity?
- What Does Drive Long-Run Biodiversity Change?
- How Does the United Nations Deal With Biodiversity?
- What Factors Affect Biodiversity?
- How Are Timber Harvesting and Biodiversity Managed in Uneven-Aged Forests?
- When Should Biodiversity Tenders Contract on Outcomes?
- Who Cares About Biodiversity?
- Why Can Financial Incentives Destroy Economically Valuable Biodiversity in Ethiopia?
- What Factors Affect an Area’s Biodiversity?
- In What Ways Is Biodiversity Economically Valuable?
- Which Human Activities Threaten Biodiversity?
- How Can Biodiversity Be Protected?
- In What Ways Is Biodiversity Ecologically Value?
- In Which Countries Is Biodiversity Economically Valuable?
- Does Species Diversity Follow Any Patterns?
- How Is Biodiversity Measured?
- What Is a Biodiversity Hotspot?
- Ecosystem Essay Topics
- Climate Change Titles
- Environment Research Topics
- Disaster Essay Titles
- Evolution Topics
- Glaciers Topics
- Hunting Questions
- Global Warming Essay Titles
- Chicago (A-D)
- Chicago (N-B)
IvyPanda. (2024, February 22). 133 Biodiversity Topics & Examples. https://ivypanda.com/essays/topic/biodiversity-essay-topics/
"133 Biodiversity Topics & Examples." IvyPanda , 22 Feb. 2024, ivypanda.com/essays/topic/biodiversity-essay-topics/.
IvyPanda . (2024) '133 Biodiversity Topics & Examples'. 22 February.
IvyPanda . 2024. "133 Biodiversity Topics & Examples." February 22, 2024. https://ivypanda.com/essays/topic/biodiversity-essay-topics/.
1. IvyPanda . "133 Biodiversity Topics & Examples." February 22, 2024. https://ivypanda.com/essays/topic/biodiversity-essay-topics/.
Bibliography
IvyPanda . "133 Biodiversity Topics & Examples." February 22, 2024. https://ivypanda.com/essays/topic/biodiversity-essay-topics/.
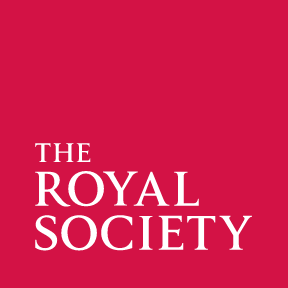
Why is biodiversity important?
Biodiversity is essential for the processes that support all life on Earth, including humans. Without a wide range of animals, plants and microorganisms, we cannot have the healthy ecosystems that we rely on to provide us with the air we breathe and the food we eat. And people also value nature of itself.
Some aspects of biodiversity are instinctively widely valued by people but the more we study biodiversity the more we see that all of it is important – even bugs and bacteria that we can’t see or may not like the look of. There are lots of ways that humans depend upon biodiversity and it is vital for us to conserve it. Pollinators such as birds, bees and other insects are estimated to be responsible for a third of the world’s crop production. Without pollinators we would not have apples, cherries, blueberries, almonds and many other foods we eat. Agriculture is also reliant upon invertebrates – they help to maintain the health of the soil crops grow in. Soil is teeming with microbes that are vital for liberating nutrients that plants need to grow, which are then also passed to us when we eat them. Life from the oceans provides the main source of animal protein for many people.
Trees, bushes and wetlands and wild grasslands naturally slow down water and help soil to absorb rainfall. When they are removed it can increase flooding. Trees and other plants clean the air we breathe and help us tackle the global challenge of climate change by absorbing carbon dioxide. Coral reefs and mangrove forests act as natural defences protecting coastlines from waves and storms.
Many of our medicines, along with other complex chemicals that we use in our daily lives such as latex and rubber, also originate from plants. Spending time in nature is increasingly understood to lead to improvements in people’s physical and mental health. Simply having green spaces and trees in cities has been shown to decrease hospital admissions, reduce stress and lower blood pressure.
Further reading
Plural valuation of nature matters for environmental sustainability and justice by Berta Martin-Lopez, Social-Ecological Systems Institute, Faculty of Sustainability, Leuphana University of Lüneburg, Germany
Climate change and biodiversity
Human activities are changing the climate. Science can help us understand what we are doing to habitats and the climate, but also find solutions.
Email updates
We promote excellence in science so that, together, we can benefit humanity and tackle the biggest challenges of our time.
Subscribe to our newsletters to be updated with the latest news on innovation, events, articles and reports.
What subscription are you interested in receiving? (Choose at least one subject)
- Skip to Page Content
- Skip to Navigation
- Skip to Search
- Skip to Footer
- COVID-19 Health and Safety
- Admissions and Ticketing
- Temporary Hall Closures
- Accessibility
- Field Trips
- Adult Group Visits
- Guided Tours
- Transportation
- Davis Family Butterfly Vivarium
- Invisible Worlds: Immersive Experience
- The Secret World of Elephants
- Turtle Odyssey
- Worlds Beyond Earth: Space Show
- Extinct and Endangered: Insects in Peril
- Grounded by Our Roots
- Ice Cold: An Exhibition of Hip-Hop Jewelry
- Opulent Oceans
- What's in a Name?
- Children & Family Programs
- Teen Programs
- Higher Education
- Adult Programs
- Educator Programs
- Evaluation, Research, & Policy
- Master of Arts in Teaching
- Online Courses for Educators
- Urban Advantage
- Viruses, Vaccines, and COVID-19
- The Science of COVID-19
- OLogy: The Science Website for Kids
- News & Blogs
- Science Topics
- Margaret Mead Festival
- Origami at the Museum
- Astrophysics
- Earth and Planetary Sciences
- Herpetology
- Ichthyology
- Ornithology
- Richard Gilder Graduate School
- Hayden Planetarium
- Center for Biodiversity and Conservation
- Institute for Comparative Genomics
- Southwestern Research Station
- Research Library
- Darwin Manuscripts Project
- Microscopy and Imaging Facility
- Science Conservation
- Computational Sciences
- Staff Directory
- Scientific Publications
- Ways to Donate
- Membership FAQ
- Benefit Events
- Corporate Support
- Planned Giving
- In memoriam: Dr. Eleanor Sterling
What Is Biodiversity?
- Podcasts and Video
- Machine Learning for Conservation
- DotDotGoose
- Conservation Biogeography
- Evidence-informed Conservation
- Integrating Biocultural Knowledge into American Samoa Mangrove Restoration and Conservation
- Biocultural Approaches to Wine Production in France
- Implementing Culturally Attuned Monitoring and Reporting Indicators
- Supporting Resilient Biocultural Land- and Seascapes in the Solomon Islands
- Community Conservation Areas
- Understanding Drivers of Wildlife Trade in Vietnam
- Past Projects
- Mangrove Ecology and Restoration in American Samoa
- Developing a Regional Conservation Strategy for Flamingos in the Americas
- Impact of Human Activity on Black Bear Habitats in the Western Great Basin
- Ecology, Evolution, and Conservation of Primates in Southeast Asia
- Understanding the Role of Palmyra Atoll for Sea Turtles in the Pacific
- Monitoring Landscapes for Conservation Planning
- Building Conservation Capacity in Vietnam
- Advancing Conservation Education
- Developing Global Capacity
- Inclusive Conservation Community Initiative (ICON)
- SCCS-NY Application & Registration
- SCCS-NY Plenaries
- SCCS-NY Code of Conduct
- SCCS-NY Workshops
- SCCS-NY 2023
- SCCS-NY 2022
- SCCS-NY 2021
- SCCS-NY 2020
- SCCS-NY 2019
- SCCS-NY 2018
- SCCS-NY 2017
- SCCS-NY 2016
- SCCS-NY 2015
- SCCS-NY 2014
- SCCS-NY 2013
- SCCS-NY 2012
- SCCS-NY 2010
- SCCS-NY 2011
- Conservation Teaching and Learning Studios
- 2019 Indicator Gathering
- Indicators of Well-being Webinar Series
- 2018 Action Group on Knowledge Systems and Indicators of Wellbeing
- Collaborative Networks
- 2015 GBIF Frontiers of Biodiversity Informatics and Modelling Species Distributions
- 2013 Milstein Science Symposium
- 2009 Exploring the Dynamic Relationship Between Health and the Environment
- 2008 Sustaining Cultural and Biological Diversity in a Rapidly Changing World: Lessons for Global Policy
- 1997-2007 Archive
- Open Source Software and Scripts
- Remote Sensing Interactives
- Lessons in Conservation: Volume 13
- Lessons in Conservation: Volume 12
- Lessons in Conservation: Volume 11
- Lessons in Conservation: Volume 10
- Lessons in Conservation: Volume 9
- Lessons in Conservation: Volume 8
- Lessons in Conservation: Volume 7
- Lessons in Conservation: Volume 6
- Lessons in Conservation: Volume 5
- Lessons in Conservation: Volume 4
- Lessons in Conservation: Volume 3
- Lessons in Conservation: Volume 2
- Lessons in Conservation: Volume 1
- Nature-Culture Indicators and Knowledge Systems Resource Directory
- Conservation Leadership Capacity Building: a Landscape Study
- Nature in Fragments: The Legacy of Sprawl
- Engaging and Learning for Conservation: Workshop on Public Participation in Scientific Research
- Fully-Protected Marine Reserves for the Future of Our Oceans
- Remote Sensing for Ecology and Conservation: A Handbook for Techniques
- For Educators
- Freshwater Mussels of the New York Metropolitan Region
- Remote Sensing Guides
- Learn More About Biodiversity
- Green Your Holidays
- Protecting Nature In Your Community
- Healthy Eating For You and the Planet: Avoiding Pesticides in Produce
- Healthy Eating for You and the Planet: Select Seasonally
- Living With Biodiversity Series
- Living With Nature: Cooking for Biodiversity
- CBC Progress Reports
- Staff Publications
- Exhibitions Created in Conjunction with the CBC
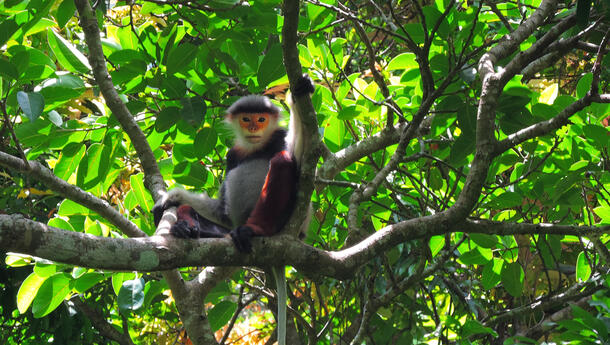
Biodiversity includes not only species we consider rare, threatened, or endangered but also every living thing—from humans to organisms we know little about, such as microbes, fungi, and invertebrates.
At the Center for Biodiversity and Conservation, we include humans and human cultural diversity as a part of biodiversity. We use the term “ biocultural ” to describe the dynamic, continually evolving and interconnected nature of people and place, and the notion that social and biological dimensions are interrelated. This concept recognizes that human use, knowledge, and beliefs influence, and in turn are influenced, by the ecological systems of which human communities are a part. This relationship makes all of biodiversity, including the species, land and seascapes, and the cultural links to the places where we live—be right where we are or in distant lands—important to our wellbeing as they all play a role in maintaining a diverse and healthy planet.
How do we study biodiversity?
Exploration and monitoring.
To study biodiversity, scientists conduct expeditions to survey and monitor species, habitats, and their interactions. On these expeditions, scientists ask questions about, measure, and collect data on various dimensions, such as population sizes and trends, distribution and habitat use, and impacts of management or other human activities. From primates in Southeast Asia to flamingos in the Andes, the CBC is engaged in numerous monitoring projects across the globe.
Tools of the trade
Biodiversity scientists use a variety of tools for collecting and analyzing data at various scales. Landscape monitoring techniques , for instance, use imaging systems such as remote sensing and drones to capture images across an area. Machine learning can be used to identify and count species or classify landscape types captured in these images or in video or audio clips. Mathematical modeling with software such as Maxent enables scientists to model species niches and distributions across these landscapes and predict how they will respond to climate change. New technological advances enhance our ability to monitor biodiversity and implement conservation and management activities.
Read more about the CBC’s Biodiversity Informatics Program to learn how information technology can be used to collect, organize, and analyze biodiversity data.

Synthesizing evidence
The vast knowledge collected through these various methods forms the evidence that decision-makers need to enact effective and sustainable conservation approaches.
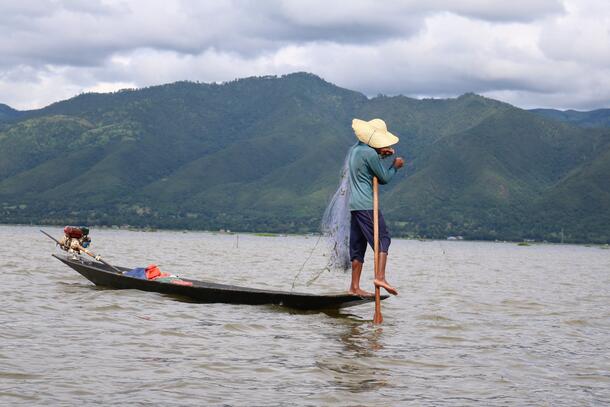
Learn more about our evidence-informed practice by reading about the CBC’s Evidence Initiative .
Building capacity
By strengthening the ability of community leaders, educators, managers, and other professionals to study biodiversity, we improve our ability to effectively manage and conserve the variety of life.
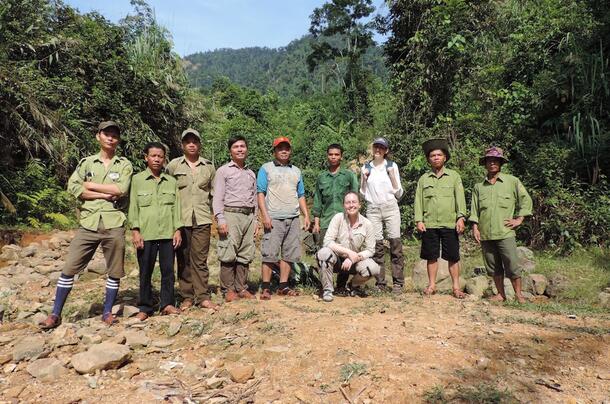
The CBC’s program in Southeast Asia develops capacity for conservation science through multidisciplinary research training of Vietnames graduate and undergraduate students, direct training of protected area staff in survey techniques, and co-leading training workshops on improving wildlife trade management.
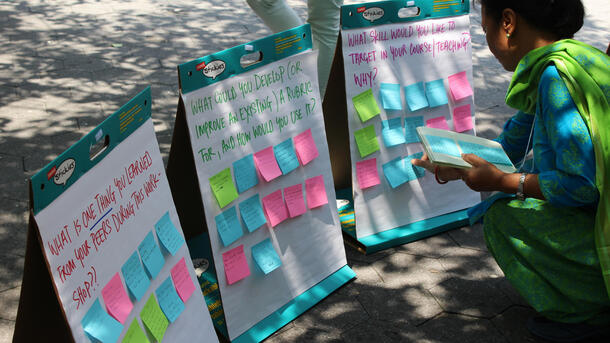
The CBC’s Network of Conservation Educators and Practitioners (NCEP) improves training, teaching, and learning in biodiversity conservation with up-to-date, open-access resources for teaching and learning on a range of conservation topics, and by leading training and research initiatives to advance the field of conservation education.
Why is biodiversity important?
Biodiversity is important to most aspects of our lives. We value biodiversity for many reasons, some utilitarian, some intrinsic. This means we value biodiversity both for what it provides to humans, and for the value it has in its own right. Utilitarian values include the many basic needs humans obtain from biodiversity such as food, fuel, shelter, and medicine. Further, ecosystems provide crucial services such as pollination, seed dispersal, climate regulation, water purification, nutrient cycling, and control of agricultural pests. Biodiversity also holds value for potential benefits not yet recognized, such as new medicines and other possible unknown services. Biodiversity has cultural value to humans as well, for spiritual or religious reasons for instance. The intrinsic value of biodiversity refers to its inherent worth, which is independent of its value to anyone or anything else. This is more of a philosophical concept, which can be thought of as the inalienable right to exist. Finally, the value of biodiversity can also be understood through the lens of the relationships we form and strive for with each other and the rest of nature. We may value biodiversity because of how it shapes who we are, our relationships to each other, and social norms. These relational values are part of peoples’ individual or collective sense of wellbeing, responsibility for, and connection with the environment. The different values placed on biodiversity are important because they can influence the conservation decisions people make every day.
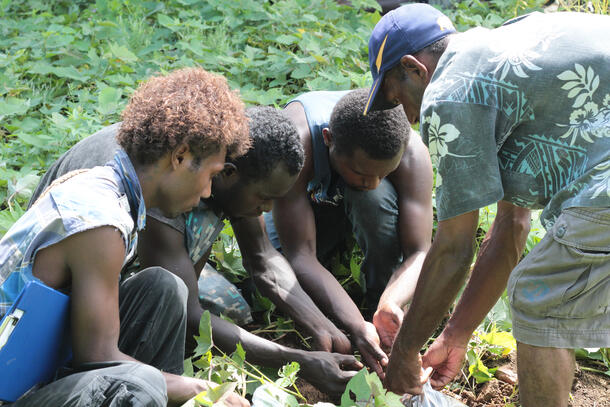
Developing community-based partnerships are crucial for supporting communities in the management and conservation of biodiversity that are vital to their wellbeing.
Threats to Biodiversity
Over the last century, humans have come to dominate the planet, causing rapid ecosystem change and massive loss of biodiversity across the planet. This has led some people to refer to the time we now live in as the “anthropocene.” While the Earth has always experienced changes and extinctions, today they are occurring at an unprecedented rate. Major direct threats to biodiversity include habitat loss and fragmentation, unsustainable resource use, invasive species, pollution, and global climate change. The underlying causes of biodiversity loss, such as a growing human population and overconsumption are often complex and stem from many interrelated factors.
The good news is that it is within our power to change our actions to help ensure the survival of species and the health and integrity of ecological systems. By understanding threats to biodiversity, and how they play out in context, we can be best prepared to manage conservation challenges. The conservation efforts of the last decades have made a significant difference in the state of biodiversity today. Over 100,000 protected areas—including national parks, wildlife refuges, game reserves, and marine protected areas, managed both by governments and local communities—provide habitat for wildlife, and help keep deforestation in check. Other types of conservation actions such as restoration, reintroduction, and the control of invasive species, have also had positive impacts on conservation efforts And these efforts have been bolstered by continuous efforts to improve environmental policies at local, regional, and global scales. It is vitally important that these policies recognize and center local values, needs, and realities to sustainably manage resources for healthy ecological as well as human communities. By acknowledging the interconnections and feedbacks between people and nature, assessing our existing knowledge, and applying evidence to our conservation decisions, we can develop effective approaches for conservation and sustainability for all life on Earth.
Learn more about what we do and what you can do to make a difference today.
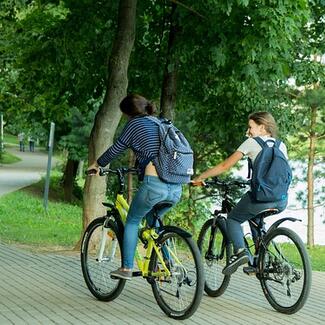
Biodiversity Heritage Library

Evolution and the Diversity of Life: Selected Essays by Ernst Mayr
Raikow, Robert J.
Date of Publication
Original publication.
The Wilson bulletin
Series / Issue
Contributed by, published by.
Columbus, Ohio, Wilson Ornithological Society, 1894-
Identifiers
BioStor: https://biostor.org/reference/200215 JSTOR: 4160928
Download MODS
We’re fighting to restore access to 500,000+ books in court this week. Join us!
Internet Archive Audio
- This Just In
- Grateful Dead
- Old Time Radio
- 78 RPMs and Cylinder Recordings
- Audio Books & Poetry
- Computers, Technology and Science
- Music, Arts & Culture
- News & Public Affairs
- Spirituality & Religion
- Radio News Archive
- Flickr Commons
- Occupy Wall Street Flickr
- NASA Images
- Solar System Collection
- Ames Research Center
- All Software
- Old School Emulation
- MS-DOS Games
- Historical Software
- Classic PC Games
- Software Library
- Kodi Archive and Support File
- Vintage Software
- CD-ROM Software
- CD-ROM Software Library
- Software Sites
- Tucows Software Library
- Shareware CD-ROMs
- Software Capsules Compilation
- CD-ROM Images
- ZX Spectrum
- DOOM Level CD

- Smithsonian Libraries
- FEDLINK (US)
- Lincoln Collection
- American Libraries
- Canadian Libraries
- Universal Library
- Project Gutenberg
- Children's Library
- Biodiversity Heritage Library
- Books by Language
- Additional Collections
- Prelinger Archives
- Democracy Now!
- Occupy Wall Street
- TV NSA Clip Library
- Animation & Cartoons
- Arts & Music
- Computers & Technology
- Cultural & Academic Films
- Ephemeral Films
- Sports Videos
- Videogame Videos
- Youth Media
Search the history of over 866 billion web pages on the Internet.
Mobile Apps
- Wayback Machine (iOS)
- Wayback Machine (Android)
Browser Extensions
Archive-it subscription.
- Explore the Collections
- Build Collections
Save Page Now
Capture a web page as it appears now for use as a trusted citation in the future.
Please enter a valid web address
- Donate Donate icon An illustration of a heart shape
Evolution and the diversity of life : selected essays
Bookreader item preview, share or embed this item, flag this item for.
- Graphic Violence
- Explicit Sexual Content
- Hate Speech
- Misinformation/Disinformation
- Marketing/Phishing/Advertising
- Misleading/Inaccurate/Missing Metadata
some of the pages have markings
![[WorldCat (this item)] [WorldCat (this item)]](https://archive.org/images/worldcat-small.png)
plus-circle Add Review comment Reviews
294 Previews
5 Favorites
Better World Books
DOWNLOAD OPTIONS
No suitable files to display here.
EPUB and PDF access not available for this item.
IN COLLECTIONS
Uploaded by station04.cebu on June 20, 2019
SIMILAR ITEMS (based on metadata)
- Open access
- Published: 09 August 2024
Creating large-scale genetic diversity in Arabidopsis via base editing-mediated deep artificial evolution
- Xiang Wang 1 na1 ,
- Wenbo Pan 2 na1 ,
- Chao Sun 3 , 4 na1 ,
- Hong Yang 2 na1 ,
- Zhentao Cheng 2 na1 ,
- Fei Yan 1 ,
- Guojing Ma 1 ,
- Yun Shang 2 ,
- Rui Zhang 3 ,
- Caixia Gao 3 , 4 ,
- Lijing Liu 1 &
- Huawei Zhang ORCID: orcid.org/0000-0002-8893-1429 2
Genome Biology volume 25 , Article number: 215 ( 2024 ) Cite this article
Metrics details
Base editing is a powerful tool for artificial evolution to create allelic diversity and improve agronomic traits. However, the great evolutionary potential for every sgRNA target has been overlooked. And there is currently no high-throughput method for generating and characterizing as many changes in a single target as possible based on large mutant pools to permit rapid gene directed evolution in plants.
In this study, we establish an efficient germline-specific evolution system to screen beneficial alleles in Arabidopsis which could be applied for crop improvement. This system is based on a strong egg cell-specific cytosine base editor and the large seed production of Arabidopsis , which enables each T1 plant with unedited wild type alleles to produce thousands of independent T2 mutant lines. It has the ability of creating a wide range of mutant lines, including those containing atypical base substitutions, and as well providing a space- and labor-saving way to store and screen the resulting mutant libraries. Using this system, we efficiently generate herbicide-resistant EPSPS, ALS, and HPPD variants that could be used in crop breeding.
Conclusions
Here, we demonstrate the significant potential of base editing-mediated artificial evolution for each sgRNA target and devised an efficient system for conducting deep evolution to harness this potential.
Genetic diversity, e.g., variation in coding sequences, non-coding genes, and expression-regulating elements, is the basis for plant domestication and crop breeding. Natural variation and random mutations induced by physical and chemical treatments are the sources of genetic diversity in conventional breeding [ 1 ]. However, with the growing global population and increasing food demand, there is an urgent need for even greater genetic diversity in modern agriculture. Fortunately, genome editing tools offer a promising solution for protein evolution [ 1 ]. There are two major ways to achieve directed evolution, insertions/deletions (indels), and base changes [ 2 , 3 , 4 , 5 ]. Indels normally disrupt the function of genes and regulatory elements. Base changes generate a wider range of genetic variants than indels, including gain-of-function changes that fine-tune gene function. Prime editor (PE) and base editor (BE) are two major editing tools for introducing targeted base substitutions [ 6 ].
PE is a “search-and-replace” technology [ 7 ]. It has been used to create a large number of base substitutions at six conserved residues of acetyl-coenzyme A carboxylase 1 ( OsACC1) , generating 16 herbicide-resistant alleles in rice [ 5 ]. However, the editing products of PE are determined by the editing templates in the pegRNAs, and one pegRNA can only generate one editing product [ 7 ]. Hence, PE is suitable for modifying a defined base at a critical position rather than generating large mutant pools of altered bases for high-throughput screening for putative critical positions.
Unlike PE, BE can generate multiple types of editing products at each sgRNA target. Thus, BE has shown greater potential than PE for generating ample base variations. Previously, BE-mediated gene artificial evolution focused on designing multiple sgRNAs to cover whole gene or key regions of these genes [ 2 ]. However, the enormous evolutionary potential at every single sgRNA site has been overlooked. For example, cytosine base editor (CBE) is capable of converting multiple cytosines within the editing window in a single mutant derivative [ 8 ]. In addition to the primary C-to-T conversion, CBE produces atypical base substitutions such as C-to-G and C-to-A, albeit at lower frequencies [ 9 ]. As a result, it can theoretically generate a vast number of alleles, with many variants appearing rarely in nature. Previous studies have obtained herbicide-resistant rice by designing multiple sgRNA targets to cover key positions of genes such as acetolactate synthase ( ALS ) using base editors [ 2 ], but this approach requires obtaining a large number of rice mutant plants, which is time and labor-consuming and low-throughput, and this method has a major penalty that the number of nucleotide and protein mutation forms obtained by every single target is thin, which limits the possibilities of evolution. The generation of large-scale genetic variation depends on having a sufficient number of mutant lines, which is hard to create in crops. Moreover, storage and phenotypic screening of large-scale mutant libraries require much space, time, and labor. So, despite the rapid development of genome edit toolbox, there are no such systems capable of large-scale targeted gene mutagenesis and high-throughput screening in crops.
Arabidopsis ( Arabidopsis thaliana ) is a model plant that is small, has a short life cycle, and produces thousands of small seeds per plant. We can conduct phenotypic screening on young Arabidopsis seedlings in Petri dishes or soil. Furthermore, it shares many essential genes with crop plants, e.g., genes regulating stress tolerance, herbicide resistance, and photosynthetic efficiency [ 10 , 11 ]. It would be a breakthrough to generate sufficient mutant derivatives of each sgRNA target required for high-throughput directed evolution in Arabidopsis to produce beneficial allelic forms for crop trait improvement. Here, we developed an efficient pipeline for germline-specific evolution that can easily produce and screen thousands to millions of mutant lines. Our studies of large mutant population produced in this way achieved significant improvements in directed evolution of genes and offered genetic resources for herbicide resistant trait and more importantly ensuing successful transmission application in crop plants. We anticipate that this approach will speed up many aspects of directed evolution for crop improvement.
Development of the germline-specific evolution system in Arabidopsis
Base editor has great potential for generating ample base variations. Due to the advantages we mentioned above, Arabidopsis might be an excellent candidate for base editor mediated directed evolution in situ owing to its advantages of high-throughput. However, the diversity of mutation generated by base editor in Arabidopsis still needs to be improved. Thus, we developed a germline-specific evolution system, which combined an efficient germline-specific base editor with next generation screening, aimed to break this hurdle and generate diverse mutant forms during sexual propagation, enabling high-throughput screening for beneficial derivatives which could be further used for crop improvement (Fig. 1 a). Base substitutions in some alleles often fail to be generated in the T1 plants probably due to the short time expression after floral dip. However, the same wild-type (WT) alleles continue to be subjected to editing in the germline cells of T1 plants during sexual propagation, thereby generating a variety of base edited lines in the T2 generation (Additional file 1 : Fig. S1). A single T1 Arabidopsis plant with an unchanged WT allele has the potential to produce thousands of seeds, approximately 75% or more of which contain T-DNA expressing the base editor. Thus, this system enables a single T1 plant with WT allele to generate thousands of independent T2 mutant lines. Compared with traditional methods that screen the T1 plants, the system built here has the potential to increase the number of mutant lines by several orders of magnitude.
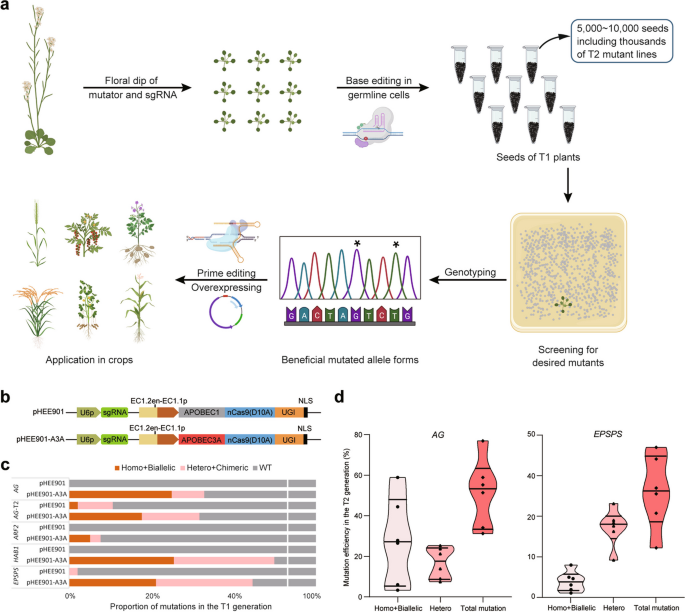
Overview of the germline-specific evolution system. a The germline-specific evolution strategy. Base editors controlled by the germline specific promoter are transformed into Arabidopsis via Agrobacterium -mediated floral dip. Base editing of the WT alleles can take place in germline cells of T1 transgenic plants during sexual reproduction. Typically, 5000 to 10,000 seeds can be collected from each T1 plant, which may contain thousands of T2 mutant lines with diverse allelic forms. The T2 lines are screened on selective plates for the desired mutants, which are subsequently genotyped to identify their mutant alleles. The desired alleles can then be used for crop breeding. b Structures of pHEE901 and pHEE901-A3A. U6p, Arabidopsis U6 promoter; EC1.2en-EC1.1p, EC1.1 promoter fused with the EC1.2 enhancer; UGI, uracil DNA glycosylase inhibitor; NLS, nuclear localization signal. c Percentages of different mutation types at five endogenous target sites created using pHEE901 and pHEE901-A3A in the T1 generation. d Frequencies of different mutation types in T2 plants from six T1 WT plants containing sgRNAs targeting AG and EPSPS , respectively. Homo, homozygous; Hetero, heterozygous
The widely used CBE vector in Arabidopsis , pHEE901, contains the rat APOBEC1-based BE3 (base editor 3) under the control of the EC1.2en-EC1.1p promoter [ 12 ]. It has been reported that unedited T1 Arabidopsis plants containing this vector can generated base edited T2 mutants. However, the editing efficiency is less than 10%, and no C-to-A/G mutant has been identified which significantly restricts the diversity of potential allele forms [ 12 ]. To address this problem, we replaced rat APOBEC1 with a more efficient cytosine deaminase, human APOBEC3A [ 13 ], to create pHEE901-A3A (Fig. 1 b). To check whether the editing efficiency of pHEE901-A3A was increased relative to that of the original BE3, we designed five sgRNAs for four target genes including agamous (AG ) [ 14 ], auxin response factor 2 ( ARF2 ) [ 15 ], 5-enolpyruvylshikimate-3-phosphate synthase ( EPSPS ) [ 16 ], and hypersensitive to ABA 1 ( HAB1 ) [ 17 ] (Additional file 1 : Figs. S2, S3 and S4). A total of 817 transgenic lines were generated, and Hi-TOM sequencing was used to assess the genotypes of all T1 plants [ 18 ]. pHEE901-A3A proved to be more efficient than pHEE901, with a wide editing window (Fig. 1 c, Additional file 2 : Tables S1 and S2). Notably, only pHEE901-A3A produced C-to-A and C-to-G conversions (Additional file 2 : Table S2).
Although pHEE901-A3A performed base editing efficiently in T1 plants, 50.0–92.3% of these plants were WT, and 2.6–14.0% were heterozygotes containing an intact WT allele (Additional file 2 : Table S1). To evaluate the editing efficiency of pHEE901-A3A during the sexual reproduction process from T1 to T2, we randomly selected six T1 WT plants containing sgRNA targeting AG and six targeting EPSPS . We then assessed the genotype of approximately 300 T2 plants from each of the T1 plants. Base editing had occurred in 31.2–76.9% of the T2 plants with sgRNA targeting AG and 14.9–45.8% of the T2 plants with sgRNA targeting EPSPS (Fig. 1 d and Additional file 2 : Table S3). These results confirmed that thousands of T2 mutant lines could be generated from a single T1 WT plant using this germline-specific evolution system.
Diverse T2 mutants can be generated at each sgRNA target using the germline-specific evolution system
To maximize the mutation depth of every single target, we investigated whether the T2 mutants were more extensive and diverse genetic variations than the T1 mutants at each target. Both protospacers of the AG and EPSPS targets contained seven cytosines (Additional file 1 : Fig. S2). In theory, all seven cytosines could be altered; we could expect to obtain lots of mutated allele forms for each target. In the event, we respectively identified 106 and 36 forms of mutated allele for AG and EPSPS in the tested 1800 T2 plants, compared with only 22 and 12 in the 88 and 85 T1 plants (Fig. 2 a, b, Additional file 1 : Figs. S4 and S5). Our findings provide strong evidences supporting a correlation between the scale of mutants and types of genetic variants. More importantly, in this experiment, we have only tested a very small number of T2 plants, equivalent to only approximately a quarter of seeds produced by one T1 plant, whereas if we characterized and screened the seeds produced by all the T1 transgenic plants, we would obtain significantly larger number of mutation forms.

The germline-specific evolution system speeds up DNA evolution. a Numbers of alleles actually obtained in the T1 and T2 generations at AG and EPSPS targets. b Comparison of the mutated allele forms obtained in the T1 and T2 generations. c Base substitution frequencies at each base in the T1 and T2 generations. Numbers above the letters indicate positions of these bases in the protospacer. PAM sequences are indicated. d Numbers of mutated allele forms with typical C-to-T changes and atypical base substitutions in the T2 generation. e Numbers of mutated allele forms in the T2 generation with their frequencies. The mutated allele forms are shown in Additional file 1 : Fig. S5. The frequencies denote the numbers of mutants harboring corresponding allele among the total plants sequenced
Analysis of the profiles of the editing products revealed that our system expanded the size of the editing window (Fig. 2 c, Additional file 1 : Figs. S4 and S5). For instance, the editing window of the EPSPS target expanded to 34 bp (from position − 18 to 16) in the T2 generation compared to 7 bp (positions 5 to 11) in the T1 plants (Fig. 2 c). Similarly, base substitutions outside of the protospacer of the AG target were detected only in the T2 plants (Fig. 2 c). We also observed that our evolution system yielded a wide range of atypical base substitutions including not only C-to-G and C-to-A but also A-to-T, G-to-A, G-to-C, and T-to-C, suggesting that deamination also occurred on A and G (Fig. 2 c). The T-to-C conversion might have been resulted from deamination of A on the complementary strand. These results underscore the significant evolutionary potential of base editing on single sgRNA target.
Although more than half of the T2 altered allele forms were the results of atypical base substitutions, the most frequent deamination events occurred on cytosines in a narrow window, and the most common change was a C-to-T transition (Fig. 2 d and Additional file 1 : Fig. S5). As a result, most of the altered allele forms, including those resulting from C-to-T conversions in the extended editing window, or involving atypical base substitutions, arose at relatively low frequencies: over 75% occurred at frequencies below 1% and more than 40% at frequencies less than 0.1% (only one plant harboring the corresponding allele in the set of approximately 1800 T2 plants) (Fig. 2 e and Additional file 1 : Fig. S5). These findings show that our system provides an effective strategy for generating large-scale mutant libraries at every single target needed for achieving high-throughput directed evolution and screening for rare mutant forms. The evolutionary depth and efficiency of atypical base substitution can be further improved by using more potent deaminases and designing multiple target sites.
The germline-specific evolution system facilitates directed evolution of proteins
Since our system increased variation at the DNA level, we expected that it would also generate a wider spectrum of variants of AG and EPSPS at the protein level. Although we observed only 2 types of full-length AG protein variant and 10 types of full-length EPSPS protein variant in T1 plants, we identified 15 and 25 forms of full-length protein, respectively, in the tested T2 plants (Fig. 3 a, b).
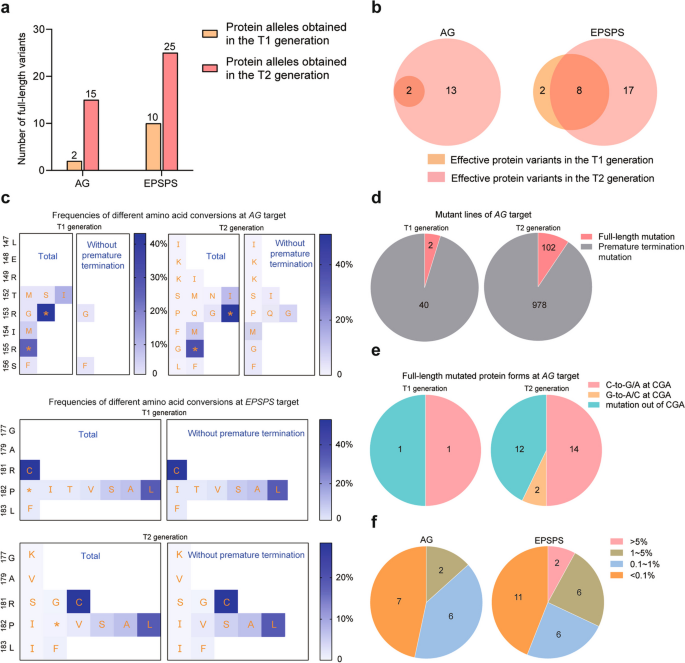
The germline-specific evolution system facilitates protein evolution. a Numbers of variants actually obtained in the T1 and T2 generations at AG and EPSPS targets. b Comparison of the altered protein forms obtained in the T1 and T2 generations. c Heatmap showing the frequencies of different amino acid substitutions in the T1 and T2 generations mutants with or without premature termination. d Numbers of plants harboring full-length mutation forms or premature stop codons in the T1 and T2 generations. e Number of mutant plants with full-length mutation variants of different types obtained in the T1 and T2 generations at the AG target. f Numbers of altered protein forms in the T2 generation with the corresponding frequencies. The altered protein forms are shown in Additional file 1 : Fig. S6. The frequencies denote the numbers of mutants harboring corresponding mutated protein forms among the total plants sequenced
Next, we analyzed variation at the amino acid level and found that the presence of atypical base substitutions led to extensive amino acid variation. For example, P182 (CCA) of EPSPS was converted not only to Ser (TCA) and Leu (TTA and CTA) but also to Ala (GCA), Val (GTA), Ile (ATA), Thr (ACA), and a stop codon (TGA) (Fig. 3 c, Additional file 1 : Figs. S5 and S6). T2 plants contained a wider variety of amino acid changes at T152, R153, I154, R155, and S156 of AG and R181 and L183 of EPSPS than T1 plants (Fig. 3 c and Additional file 1 : Fig. S6). Additionally, due to the broader editing window, amino acid changes at L147, E148, and R149 of AG and G177 and A179 of EPSPS were detected only in T2 plants (Fig. 3 c).
Premature termination of protein translation, which often results from C-to-T conversions at CAA, CGA, and CAG codons, poses a significant challenge to CBE-mediated directed evolution of proteins, as it can impact the evolution of not only these specific codons but also adjacent ones. For instance, the protospacer region of the AG target comprises two CGA codons encoding R153 and R155, with cytosines situated at positions 5 and 11 (Fig. 2 c). In the T1 generation, only two of 42 mutant lines contained full-length mutated proteins with single amino acid changes (R153G or S156F), while the others resulted in premature termination, which prevented the functional detection of variants of the other amino acids in the protospacer region (Fig. 3 d and Additional file 1 : Fig. S6). Screening a large T2 mutant library circumvented this limitation: 102 of 1080 T2 mutated alleles encoded 15 different forms of full-length mutated AG protein were created, including amino acid conversions at five different positions (Additional file 1 : Fig. S6). We noted that in these full-length AG variants, CGA either underwent atypical C-to-G/A or G-to-A/C changes instead of C-to-T changes or base editing occurred specifically on the surrounding codons but not CGA (Fig. 3 e and Additional file 1 : Fig. S7). These results showed that our evolution system is well protected against the adverse effects of mutant protein premature stoppage on artificial evolution. Similar to the frequency at the DNA level, over 60% of the different protein variants appeared at a frequency below 1% and more than 40% at a frequency less than 0.1% (Fig. 3 f). In sum, the diversity of mutation significantly increased by using this germline-specific evolution system which could enable comprehensive and large-scale directed evolution of proteins.
Screening for EPSPS mutants exhibiting herbicide-resistance
Glyphosate is one of the most widely used herbicides that target EPSPS [ 16 ]. As the targeting site of EPSPS sgRNA used above is in close proximity to the coding sequence of key amino acids related to glyphosate resistance, we assessed the potential to generate herbicide resistant mutants. We sowed approximately 50,000 T2 seedlings from 10 T1 WT plants containing this sgRNA to 1/2 MS medium supplemented with 100 mM glyphosate (Fig. 4 a). Only three seedlings grew, and all of them produced the same form of EPSPS with a rare combination of amino acid substitutions: T178I/A179V/P182S (Fig. 4 b). This variant was the result of three C-to-T substitutions on C-4, C-1, and C8 and was not encountered in the previously sequenced T2 plants (Fig. 4 b and Additional file 1 : Fig. S5). Moreover, C-4 was not changed in any of the previously sequenced T2 plants (Fig. 2 c). These results demonstrate that a large mutant library is critical for isolating rare beneficial mutants. Interestingly, the T178I/A179V/P182S form of EPSPS has been found in a naturally occurring high glyphosate-resistant strain of herbicide-resistant weed, and its equivalent variants have been used in rice and maize breeding [ 16 , 19 , 20 ].
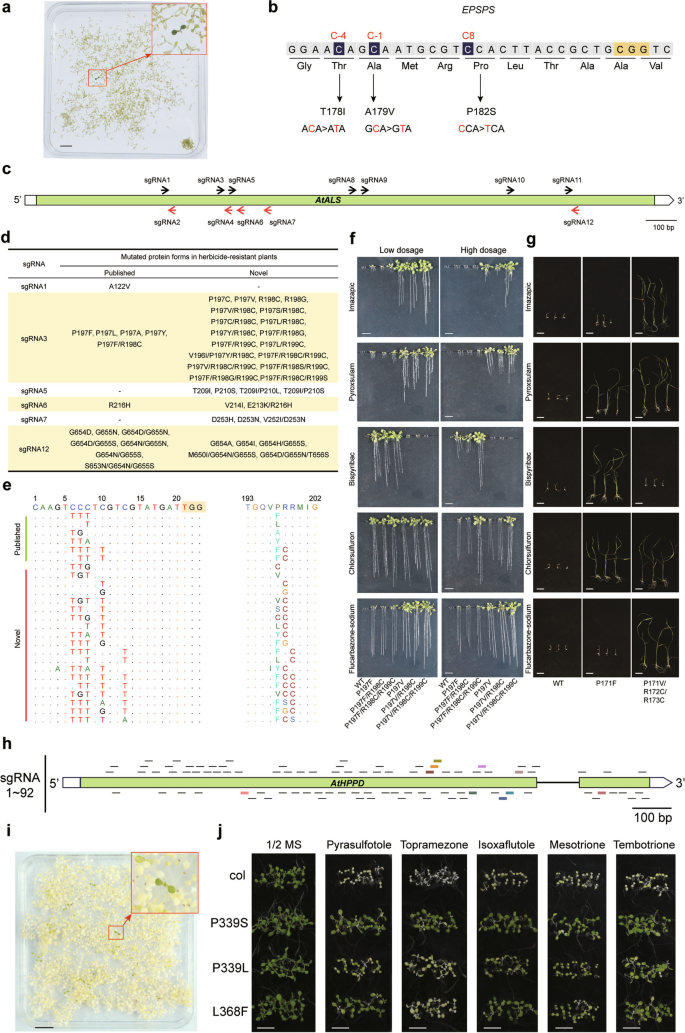
Generating herbicide resistant mutants with the germline-specific evolution system. a Representative glyphosate-resistant EPSPS mutant created by this evolution system. Scale bar, 1 cm. b The base substitutions in the glyphosate-resistant seedlings shown in a . c Schematic of the ALS gene. White and green bars represent untranslated regions and exons respectively. The arrows indicate the positions of the sgRNAs on the sense (black rightward arrows) and antisense (red leftward arrows) strands. d Amino acid changes in the herbicide-resistant ALS mutants. e Base substitutions in the herbicide-resistant ALS mutants generated by sgRNA3. Numbers above the bases indicate positions in the protospacer, or positions of the amino acids in the protein. The PAM sequence is marked by yellow shadow. Dots indicate unmutated nucleotides and unchanged amino acids. f Representative Arabidopsis seedlings of WT and ALS mutants harboring P197F, P197F/R198C, P197F/R198C/R199C, P197V, P197V/R198C, and P197V/R198C/R199C mutations grown on 1/2 MS plates supplemented with different concentrations and types of ALS-targeting herbicides for 2 weeks. Scale bar, 1 cm. g Representative rice plants with WT, genome edited mutants with P171F and P171V/R172C/R173C forms of OsALS1, grown on medium with different ALS-targeting herbicides for 16 days. Scale bar, 2 cm. The herbicide concentrations used in these treatments are given in the “ Methods ” section. h Schematic of the HPPD gene and its sgRNAs used in this study. White and green bars represent untranslated regions and exons respectively, and lines represent introns. The lines above or below the schematic represent sgRNAs, with bold and colored ones indicate sgRNAs that resulted in herbicide-resistant mutant forms. i Representative topramezone-resistant HPPD mutant. Scale bar, 1 cm. j Representative Arabidopsis seedlings of WT and HPPD mutants harboring P339S, P339L, and L368F mutations grown on 1/2 MS plates supplemented with different concentrations and types of HPPD-targeting herbicides for 9 days. Scale bar, 1 cm
In the above screening, 10 unedited T1 WT plants generated more than 50,000 T2 seeds that could be easily accommodated in 10 Eppendorf tubes. According to the mutation rate calculated above, these T2 seeds should contain approximately 15,000 independent mutant lines, a number that is almost impossible to obtain in other ways. Moreover, we needed only 25 petri dishes to screen for herbicide-resistance for the 50,000 seeds. Thus, the use of the germline-specific evolution system in Arabidopsis is space/labor-saving and effective in generating, storing and high-throughput screening of mutant libraries.
Screening for herbicide-resistant ALS mutants
We also conducted directed evolution of Arabidopsis ALS, the target of five groups of commercial herbicides: imidazolinones (IMI), pyrimidinylthiobenzoates (PTB), sulfonylaminocarbonyltriazolinones (SCT), sulfonylureas (SU), and triazolopyrimidines (TP) [ 21 ]. The development of ALS mutants with broad-spectrum resistance (BSR) to several of these herbicides could benefit agricultural breeding focused on weed control. To isolate such a mutant, we designed 12 sgRNAs targeting the coding sequences of conserved ALS regions between Arabidopsis and rice and then created more than 60 transgenic T1 plants for each sgRNA (Fig. 4 c, Additional file 1 : Figs. S8 and S9). To screen for herbicide-resistant mutants, we grew 120 T2 seedlings from each T1 plant on 1/2 MS plates containing chlorsulfuron (SU herbicide), bispyribac (PTB herbicide), or imazapic (IMI herbicide). In total, we screened over 100,000 T2 seedlings from 834 T1 plants, determined the genotypes of 1244 herbicide-resistant T2 plants by Hi-TOM sequencing, and identified 46 forms of ALS mutant, including 32 that had not been reported previously (Fig. 4 d, e, Additional file 1 : Fig. S10 and Additional file 2 : Table S4).
We focused on alleles with multiple resistance and found that one T2 seedling with a P197V/R198C/R199C mutation exhibited tolerance to imazapic; meanwhile, P197V and P197V/R198C mutations were observed in five chlorsulfuron-resistant seedlings (Additional file 2 : Table S4). All P197F, P197F/R198C and P197F/R198C/R199C were resistance to three herbicides, moreover, P197F/R198C and P197F/R198C/R199C mutants showed resistance to all three tested herbicides (Additional file 2 : Table S4). To confirm their resistances to different ALS-targeting herbicides, we generated T3 homozygous derivatives. None of these mutations had a significant influence on plant growth in the absence of herbicide treatment (Additional file 1 : Fig. S11). We then grew the mutants on 1/2 MS plates with each of five ALS-targeting herbicides (Fig. 4 f). P197V offered robust resistance to four tested herbicides, namely imazapic, pyroxsulam, chlorsulfuron, and flucarbazone, whereas P197F showed strong resistance only to bispyribac and chlorsulfuron (Fig. 4 f and Additional file 1 : Fig. S12). Moreover, the R198C/R199C mutation increased the tolerance of P197F/V to higher doses of several herbicides (Fig. 4 f and Additional file 1 : Fig. S12).
To check whether P171F and P197V/R198C/R199C could be used as BSR forms of ALS in crops, we generated genome edited rice plants with modified gene sequences encoding the corresponding P171F and P171V/R172C/R173C variants of OsALS1 using prime editing (Additional file 1 : Fig. S13). The BSR of both variants in rice was similar to that observed in Arabidopsis , indicating that the germline-specific evolution system works well for crop improvement (Fig. 4 g and Additional file 1 : Fig. S14). These results demonstrate that the germline-specific evolution system can perform efficient and effective directed evolution of prominent agronomic trait genes, and more importantly, the obtained mutations can be good applied in molecular breeding of other crops.
Screening for herbicide-resistant HPPD mutants
To conduct direct evolution on 4-hydroxyphenylpyruvate dioxygenase (HPPD), another conserved protein and important target of commercial herbicides (Additional file 1 : Fig. S15) [ 22 ], we generated a Cas9-NG vector [ 23 ], pHEE901-A3A-NG, to expand the target scope of our system, and designed 92 sgRNAs to cover its coding sequence (Fig. 4 h and Additional file 2 : Table S5). Over 3 million T2 seedlings were planted on 1/2 MS medium supplemented with five HPPD inhibitor herbicides, including pyrasulfotole, topramezone, mesotrione, tembotrione, and isoxaflutole (Fig. 4 i). We obtained 26 herbicide-resistant HPPD mutation forms caused by 10 sgRNAs (Fig. 4 h, Additional file 1 : Figs. S16 and S17). These mutant forms contained substitutions on 17 highly conserved amino acid residues, particularly among dicot plants (Additional file 1 : Figs. S15 and S16). Among the 26 mutant forms, 14 occurred on the target of sgRNA68, and all of them contained amino acid substitutions on P339, suggesting that the critical roles of P339 and its surrounding amino acids in herbicide binding. In a previous study, six amino acids, including P339, were identified as potentially contributory for the divergence in herbicide-binding activity between AtHPPD and ZmHPPD [ 24 ]. Our findings indicate that the diversity on this amino acid may partially account for the HPPD-type herbicide resistance observed in maize. Further testing in T2 generation indicated that mutants on the target of sgRNA68 and sgRNA73 showed higher degree of resistance to these herbicides (Fig. 4 j). However, all of these mutants have not provided enough tolerance which can be applied in crop plants. In these assays, we have identified several important regions of HPPD strongly relating to herbicide resistance. Thus, in the future, we will simultaneously express several sgRNAs to cover all of these key regions to perform larger scale evolution for creating high level BSR mutants, which can be applied in crop plants.
In this study, we describe the potential of BE-mediated artificial evolution for generating a large allelic diversity at single target which has been overlooked before, probably due to the lack of an efficient tool for producing and screening large mutant libraries. To address this issue, we established a germline-specific evolution system to screen beneficial alleles in Arabidopsis which could be applied for crop improvement. This system allows unedited T1 Arabidopsis plants with germline-specifically expressed A3A-BE to produce thousands of T2 mutant lines, all of which can be stored in a small Eppendorf tube, enabling space-saving and labor-efficient phenotypic screening.
It has been demonstrated that A3A-BE behave broader editing window than APOBEC1-mediated BE due to its higher editing efficiency [ 13 ]. Rare base substitutions, which occur at very low frequencies, can only been found in large-scale mutant pool. Thus, the use of this germline-specific evolution system broadens the editing window and permits the detection of atypical base conversions, significantly increasing the available genetic diversity at both the DNA and protein levels. Importantly, this system reduces the inhibitory effect of premature translation termination on the directed evolution of proteins. We believe that the germline-specific evolution system should also permit us to co-evolve key amino acid residues distributed across different regions of a protein.
Several in vivo evolution studies have been conducted in plants, with screenings primarily focusing on crops to identify herbicide-resistant alleles [ 2 , 25 , 26 , 27 , 28 , 29 ]. These screenings typically involved selecting calli on plates supplemented with herbicide, from which T0 resistant lines were subsequently generated. However, the labor-intensive and space-demanding nature of callus transformation limited the selection to only a few thousand calli, thus restricting the variety of mutants that could be examined. In contrast, our system conducted screening in the T2 generation of Arabidopsis , facilitating the evaluation of millions of transgenic lines for the same purpose, resulting in deep evolution on each sgRNA and higher yield of herbicide-resistant mutant alleles. For instance, in comparison to the study by Kuang et al., who employed 63 sgRNAs to evolve ALS in rice and identified only five types of herbicide-resistant variants, with P171F being the most potent [ 2 ], we discovered 46 variants. Among these, P171F/R172C/R173C and P171V/R172C/R173C exhibited stronger and broader resistance to ALS-targeting herbicides than P171F.
Multiple strategies could be applied to further increase the genetic diversity created by the germline-specific evolution system. For instance, dCas9-AID(x) has shown the capability to induce more C-to-G/A substitutions compared to dCas9-AID(x)-UGI [ 30 ]. Therefore, by modifying A3A-CBE through the removal of the UGI or replacing UGI with uracil-DNA glycosylase [ 31 ], we could potentially amplify the mutation forms. Furthermore, exploring the substitution of A3A-CBE with different glycosylase base editors to facilitate C-to-N, T-to-N, A-to-N, and G-to-N substitutions is another promising avenue [ 32 , 33 ]. Additionally, the utilization of adenine and cytosine base editor (ACBE) could enable the simultaneous base substitution of both A and C nucleotides [ 25 , 34 , 35 , 36 , 37 , 38 , 39 ]. These prospective strategies present exciting directions for our research. The use of BE-mediated evolution system is complementary to that of PE, which is well-suited to achieving directed changes at critical positions in a gene rather than high-throughput screening at the whole-gene level. Using BE, our system can identify critical positions for focused PE-mediated directed evolution.
In addition to studying resistance traits, Arabidopsis is an excellent model plant for investigating various aspects such as photosynthesis rate, fertilizer use efficiency, disease resistance, abiotic tolerance, and heavy metal absorption. Many key genes that determine these traits are highly conserved between Arabidopsis and crop plants. The germline-specific evolution system enables the generation of a diverse library of mutant lines for screening purposes. While some mutations can be easily screened in petri dishes by naked eye, certain phenotypic screenings may require more extensive work and equipment compared to screening for herbicide resistance. For instance, stresses such as salt, temperature fluctuations, pathogens, and various other stressors can lead to an increase in cytoplasmic Ca 2+ levels and a decline in photosystem II activity [ 40 , 41 , 42 ]. In such cases, we can screen for mutants affecting plant responses to these stresses by utilizing an aequorin-expressing background [ 43 , 44 ], which detects Ca 2+ signals and exhibits fluorescence, or screen for mutants with altered photosystem II activity using Imaging-PAM [ 45 ]. Anyway, the workload for screening in Arabidopsis is typically smaller than that in crop plants. Furthermore, there is potential to leverage phenomics tools in combination with image recognition using machine learning to establish a series of efficient high-throughput phenotypic screening systems.
Base editing holds significant promise for artificial evolution, but so far, its full potential has been underestimated. To unlock this potential, the availability of expansive mutant pools with diverse genetic profiles and reliable high-throughput screening systems is crucial. The germline-specific evolution system we established in this study has demonstrated the capacity to generate a wide range of genetic variations by introducing unconventional base substitutions and expanding editing window and to perform large scale screening in a space/labor-saving way. This system has successfully addressed the challenge of premature termination, a significant hurdle in CBE-mediated protein evolution. Notably, the application of this system has enabled the efficient production of herbicide-resistant variants, showcasing its potential benefits for crop breeding.
Plant material and growth conditions
Arabidopsis ecotype Columbia (Col-0) was used for transformation and phenotypic analysis. All Arabidopsis plants were grown in a growth chamber with a 22 °C, 16/8 h day/night photoperiod, and 70% relative humidity. Rice variety Zhonghua11 was used for Agrobacterium -mediated transformation of rice callus cells, as previously described [ 46 ]. Hygromycin at 50 μg/mL was used to select transgenic calli, and transgenic plantlets were regenerated on selective medium 10 ~ 12 weeks later. The transgenic plantlets were grown at 28 °C and 16/8 h day/night photoperiod in bottles.
Plasmid construction
To generate plasmid pHEE901-A3A, pHEE901 was digested with Xba I and Sac I to generate a 12.8 kb fragment, and pH-A3A-PBE plasmid was digested with Avr II and Sac I, and a 5.1-kb fragment was recovered [ 13 ]. Then, the two fragments were ligated using T4 DNA ligase to produce pHEE901-A3A.
To generate the pHEE901-A3A-NG plasmid, we firstly digested the pHEE901-A3A using Mlu I and Sac I. Next, we ligated this plasmid backbone with a 1.2-kb fragment that was digested from pHUE411-NG using the same enzymes to obtain an intermedia plasmid lacking uracil glycosylase inhibitor ( UGI) . Then, the UGI sequence was amplified from pHEE901-A3A using the primer pair UGI-F and UGI-R and inserted into the Sac I-digested intermedia plasmid by Gibson assembly to generate the final pHEE901-A3A-NG plasmid.
To obtain Arabidopsis transgenic lines, 109 pairs of oligonucleotides encoding the designed sgRNAs were synthesized, annealed, and cloned into Bsa I - digested pHEE901, pHEE901-A3A, or spCas9-NG by T4 DNA ligase. The sequences of the synthesized DNA oligos and all primers used in this study are listed in Additional file 2 : Table S5.
For the generation of genome edited rice ALS mutants, the diagram and sequence of pegRNA and nicking sgRNA are listed in Additional file 1 : Fig. S18. These sequences were synthesized and digested with Bsa I and then inserted into the Bsa I digested Prime editing vector enPPE2 [ 47 ].
Agrobacterium-mediated transformation of Arabidopsis
Constructs were transformed into Agrobacterium tumefaciens strain GV3101 and introduced into Arabidopsis by the floral dip method as described [ 48 ]. Seeds were harvested one-and-a-half months later and selected on 1/2 MS plates containing 25 μg/mL hygromycin. Resistant seedlings were transplanted to soil 2 weeks later.
Agrobacterium-mediated transformation of rice callus cells
Constructs were transformed into Agrobacterium tumefaciens strain EHA105 by electroporation. Agrobacterium -mediated transformation of Zhonghua11 callus cells was carried out as reported [ 46 ]. Three days after cocultivation, the calluses were moved to medium with 50 μg/mL hygromycin to select positive transformants.
Assessment of base editing efficiency
To measure the editing efficiency of T1 plants, samples of WT and T1 plants were collected. The editing efficiency of T2 plants was measured using samples collected from the progenies of WT T1 lines. DNA was extracted by heating samples at 95 °C for 10 min in DNA extraction buffer (50 mM Tris–HCl pH 7.5, 300 mM NaCl, 300 mM sucrose). After centrifugation at 12,000 g for 5 min, supernatants were collected and used as templates for PCR using SanTaq PCR Mix (Sangon Biotech). PCR products were sequenced by Sanger sequencing or Hi-TOM sequencing [ 18 ]. The sequencing results were analyzed using the BioEdit software.
The mutation type (homozygous, heterozygous, biallelic or chimeric) of each T1 and T2 plant was determined by Hi-TOM sequencing. Reads that represented less than 10% of the results of Hi-TOM sequencing were considered aerosol contaminants or technical errors. Plants that contained one allele were identified as WT or homozygote; plants had two alleles with within-twice frequency differences were considered to have two equal alleles, i.e., to be heterozygous or biallelic; otherwise, they were considered chimeric.
The frequencies of DNA alleles, protein variants, and single base or amino acid changes were calculated as number of plants with these changes versus the total number of plants examined.
Selection of herbicide-resistant plants
To screen the herbicide-resistant EPSPS mutants, seeds of T1 plants were sown on 1/2 MS plates supplemented with 100 mM glyphosate. To select the herbicide-resistant ALS mutants, seeds of T1 plants were planted on 1/2 MS plates containing 0.02 mg/L chlorsulfuron, 0.03 mg/L bispyribac, or 0.05 mg/L imazapic. To obtain the herbicide-resistant HPPD mutants, seeds of T1 plants were sown on 1/2 MS plates supplemented with 0.75 mg/L pyrasulfotole, 0.1 mg/L topramezone, 0.1 mg/L mesotrione, 0.1 mg/L tembotrione, or 0.04 mg/L isoxaflutole. Resistant seedlings were transplanted to soil two weeks later for DNA extraction and seed collection. Hi-TOM sequencing was used to determine the mutant alleles in herbicide-resistant plants.
BSR assay for ALS mutants
Seeds of the P197F, P197F/R198C, P197F/R198C/R199C, P197V, P197V/R198C, and P197V/R198C/R199C mutants were sown on 1/2 MS plates containing different concentrations of herbicides (low dosages: 0.04 mg/L imazapic, 0.04 mg/L pyroxsulam, 0.03 mg/L bispyribac, 0.3 mg/L chlorsulfuron or 0.3 mg/L flucarbazone-sodium; high dosages: 0.08 mg/L imazapic, 0.08 mg/L pyroxsulam, 0.06 mg/L bispyribac, 0.6 mg/L chlorsulfuron or 0.6 mg/L flucarbazone-sodium) for the BSR assay. After 2 weeks, photographs were taken, and the fresh weights of individual plants were recorded.
To investigate whether P171V/R172C/R173C, the equivalent OsALS mutant to P197V/R198C/R199C of AtALS, conferred BSR to ALS herbicides in rice, genome edited plants with modified gene sequences encoding the corresponding P171F and P171V/R172C/R173C variants of OsALS1-using prime editor in T1 rice plants were grown on 1/2 MS medium for 2 days and transferred to 1/2 MS solid medium containing 0.9 mg/L imazapic, 0.4 mg/L pyroxsulam, 1.68 mg/L bispyribac, 8 mg/L chlorsulfuron, or 3.15 mg/L flucarbazone-sodium for 16 days to check for growth.
BSR assay for HPPD mutants
Seeds of T2 of HPPD mutants were sown on 1/2 MS plates supplemented with 0.2 mg/L pyrasulfotole, 0.03 mg/L topramezone, 0.02 mg/L isoxaflutole, 0.03 mg/L mesotrione, or 0.03 mg/L tembotrione for 9 days.
Availability of data and materials
All study data are included in the article and supporting information.
Chen K, Wang Y, Zhang R, Zhang H, Gao C. CRISPR/Cas genome editing and precision plant breeding in agriculture. Annu Rev Plant Biol. 2019;70:667–97.
Article CAS PubMed Google Scholar
Kuang Y, Li S, Ren B, Yan F, Spetz C, Li X, Zhou X, Zhou H. Base-editing-mediated artificial evolution of OsALS1 in planta to develop novel herbicide-tolerant rice germplasms. Mol Plant. 2020;13:565–72.
Rodriguez-Leal D, Lemmon ZH, Man J, Bartlett ME, Lippman ZB. Engineering quantitative trait variation for crop improvement by genome editing. Cell. 2017;171(470–480): e478.
Google Scholar
Song X, Meng X, Guo H, Cheng Q, Jing Y, Chen M, Liu G, Wang B, Wang Y, Li J, Yu H. Targeting a gene regulatory element enhances rice grain yield by decoupling panicle number and size. Nat Biotechnol. 2022;40:1403–11.
Xu R, Liu X, Li J, Qin R, Wei P. Identification of herbicide resistance OsACC1 mutations via in planta prime-editing-library screening in rice. Nat Plants. 2021;7:888–92.
Anzalone AV, Koblan LW, Liu DR. Genome editing with CRISPR-Cas nucleases, base editors, transposases and prime editors. Nat Biotechnol. 2020;38:824–44.
Anzalone AV, Randolph PB, Davis JR, Sousa AA, Koblan LW, Levy JM, Chen PJ, Wilson C, Newby GA, Raguram A, Liu DR. Search-and-replace genome editing without double-strand breaks or donor DNA. Nature. 2019;576:149–57.
Article CAS PubMed PubMed Central Google Scholar
Komor AC, Kim YB, Packer MS, Zuris JA, Liu DR. Programmable editing of a target base in genomic DNA without double-stranded DNA cleavage. Nature. 2016;533:420–4.
Rees HA, Liu DR. Base editing: precision chemistry on the genome and transcriptome of living cells. Nat Rev Genet. 2018;19:770–88.
Koornneef M, Meinke D. The development of Arabidopsis as a model plant. Plant J. 2010;61:909–21.
Somerville C, Koornneef M. A fortunate choice: the history of Arabidopsis as a model plant. Nat Rev Genet. 2002;3:883–9.
Chen Y, Wang Z, Ni H, Xu Y, Chen Q, Jiang L. CRISPR/Cas9-mediated base-editing system efficiently generates gain-of-function mutations in Arabidopsis. Sci China Life Sci. 2017;60:520–3.
Zong Y, Song Q, Li C, Jin S, Zhang D, Wang Y, Qiu JL, Gao C: Efficient C-to-T base editing in plants using a fusion of nCas9 and human APOBEC3A. Nat Biotechnol. 2018;36:950–3.
Wu HW, Deng S, Xu H, Mao HZ, Liu J, Niu QW, Wang H, Chua NH. A noncoding RNA transcribed from the AGAMOUS (AG) second intron binds to CURLY LEAF and represses AG expression in leaves. New Phytol. 2018;219:1480–91.
Schruff MC, Spielman M, Tiwari S, Adams S, Fenby N, Scott RJ. The AUXIN RESPONSE FACTOR 2 gene of Arabidopsis links auxin signalling, cell division, and the size of seeds and other organs. Development. 2006;133:251–61.
Perotti VE, Larran AS, Palmieri VE, Martinatto AK, Alvarez CE, Tuesca D, Permingeat HR. A novel triple amino acid substitution in the EPSPS found in a high-level glyphosate-resistant Amaranthus hybridus population from Argentina. Pest Manag Sci. 2019;75:1242–51.
Wang Z, Ji H, Yuan B, Wang S, Su C, Yao B, Zhao H, Li X. ABA signalling is fine-tuned by antagonistic HAB1 variants. Nat Commun. 2015;6:8138.
Article PubMed Google Scholar
Liu Q, Wang C, Jiao X, Zhang H, Song L, Li Y, Gao C, Wang K. Hi-TOM: a platform for high-throughput tracking of mutations induced by CRISPR/Cas systems. Sci China Life Sci. 2019;62:1–7.
Jiang Y, Chai Y, Qiao D, Wang J, Xin C, Sun W, Cao Z, Zhang Y, Zhou Y, Wang XC, Chen QJ. Optimized prime editing efficiently generates glyphosate-resistant rice plants carrying homozygous TAP-IVS mutation in EPSPS. Mol Plant. 2022;15:1646–9.
Qiao D, Wang J, Lu MH, Xin C, Chai Y, Jiang Y, Sun W, Cao Z, Guo S, Wang XC, Chen QJ. Optimized prime editing efficiently generates heritable mutations in maize. J Integr Plant Biol. 2023;65:900–6.
Garcia MD, Nouwens A, Lonhienne TG, Guddat LW. Comprehensive understanding of acetohydroxyacid synthase inhibition by different herbicide families. Proc Natl Acad Sci U S A. 2017;114:E1091–100.
Lin HY, Dong J, Dong J, Yang WC, Yang GF. Insights into 4-hydroxyphenylpyruvate dioxygenase-inhibitor interactions from comparative structural biology. Trends Biochem Sci. 2023;48:568–84.
Nishimasu H, Shi X, Ishiguro S, Gao L, Hirano S, Okazaki S, Noda T, Abudayyeh OO, Gootenberg JS, Mori H, et al. Engineered CRISPR-Cas9 nuclease with expanded targeting space. Science. 2018;361:1259–62.
Schindler CEM, Hollenbach E, Mietzner T, Schleifer KJ, Zacharias M. Free energy calculations elucidate substrate binding, gating mechanism, and tolerance-promoting mutations in herbicide target 4-hydroxyphenylpyruvate dioxygenase. Protein Sci. 2019;28:1048–58.
Li C, Zhang R, Meng X, Chen S, Zong Y, Lu C, Qiu JL, Chen YH, Li J, Gao C. Targeted, random mutagenesis of plant genes with dual cytosine and adenine base editors. Nat Biotechnol. 2020;38:875–82.
Liu X, Qin R, Li J, Liao S, Shan T, Xu R, Wu D, Wei P. A CRISPR-Cas9-mediated domain-specific base-editing screen enables functional assessment of ACCase variants in rice. Plant Biotechnol J. 2020;18:1845–7.
Ren Q, Sretenovic S, Liu G, Zhong Z, Wang J, Huang L, Tang X, Guo Y, Liu L, Wu Y, et al. Improved plant cytosine base editors with high editing activity, purity, and specificity. Plant Biotechnol J. 2021;19:2052–68.
Wang H, He Y, Wang Y, Li Z, Hao J, Song Y, Wang M, Zhu JK. Base editing-mediated targeted evolution of ACCase for herbicide-resistant rice mutants. J Integr Plant Biol. 2022;64:2029–32.
Zhang A, Shan T, Sun Y, Chen Z, Hu J, Hu Z, Ming Z, Zhu Z, Li X, He J, et al. Directed evolution rice genes with randomly multiplexed sgRNAs assembly of base editors. Plant Biotechnol J. 2023;21:2597–610.
Ma Y, Zhang J, Yin W, Zhang Z, Song Y, Chang X. Targeted AID-mediated mutagenesis (TAM) enables efficient genomic diversification in mammalian cells. Nat Methods. 2016;13:1029–35.
Zhao D, Li J, Li S, Xin X, Hu M, Price MA, Rosser SJ, Bi C, Zhang X. Glycosylase base editors enable C-to-A and C-to-G base changes. Nat Biotechnol. 2021;39:35–40.
Tong H, Wang X, Liu Y, Liu N, Li Y, Luo J, Ma Q, Wu D, Li J, Xu C, Yang H. Programmable A-to-Y base editing by fusing an adenine base editor with an N-methylpurine DNA glycosylase. Nat Biotechnol. 2023;41:1080–4.
Ye L, Zhao D, Li J, Wang Y, Li B, Yang Y, Hou X, Wang H, Wei Z, Liu X, et al. Glycosylase-based base editors for efficient T-to-G and C-to-G editing in mammalian cells. Nat Biotechnol. 2024:1–10. https://doi.org/10.1038/s41587-023-02050-w .
Chen L, Hong M, Luan C, Gao H, Ru G, Guo X, Zhang D, Zhang S, Li C, Wu J, et al. Adenine transversion editors enable precise, efficient A*T-to-C*G base editing in mammalian cells and embryos. Nat Biotechnol. 2024;42:638–50.
Gaudelli NM, Komor AC, Rees HA, Packer MS, Badran AH, Bryson DI, Liu DR. Programmable base editing of A*T to G*C in genomic DNA without DNA cleavage. Nature. 2017;551:464–71.
Grunewald J, Zhou R, Lareau CA, Garcia SP, Iyer S, Miller BR, Langner LM, Hsu JY, Aryee MJ, Joung JK. A dual-deaminase CRISPR base editor enables concurrent adenine and cytosine editing. Nat Biotechnol. 2020;38:861–4.
Article PubMed PubMed Central Google Scholar
Sakata RC, Ishiguro S, Mori H, Tanaka M, Tatsuno K, Ueda H, Yamamoto S, Seki M, Masuyama N, Nishida K, et al. Base editors for simultaneous introduction of C-to-T and A-to-G mutations. Nat Biotechnol. 2020;38:865–9.
Xie J, Huang X, Wang X, Gou S, Liang Y, Chen F, Li N, Ouyang Z, Zhang Q, Ge W, et al. ACBE, a new base editor for simultaneous C-to-T and A-to-G substitutions in mammalian systems. BMC Biol. 2020;18:131.
Zhang X, Zhu B, Chen L, Xie L, Yu W, Wang Y, Li L, Yin S, Yang L, Hu H, et al. Dual base editor catalyzes both cytosine and adenine base conversions in human cells. Nat Biotechnol. 2020;38:856–60.
Chauhan J, Prathibha MD, Singh P, Choyal P, Mishra UN, Saha D, Kumar R, Anuragi H, Pandey S, Bose B, et al: Plant photosynthesis under abiotic stresses: Damages, adaptive, and signaling mechanisms. Plant Stress. 2023;10:100296.
McAinsh MR, Pittman JK. Shaping the calcium signature. New Phytol. 2009;181:275–94.
Pirayesh N, Giridhar M, Ben Khedher A, Vothknecht UC, Chigri F. Organellar calcium signaling in plants: an update. Biochim Biophys Acta Mol Cell Res. 2021;1868: 118948.
Alonso MT, Rodriguez-Prados M, Navas-Navarro P, Rojo-Ruiz J, Garcia-Sancho J. Using aequorin probes to measure Ca(2+) in intracellular organelles. Cell Calcium. 2017;64:3–11.
Sun S, Zhang X, Chen K, Zhu X, Zhao Y. Screening for Arabidopsis mutants with altered Ca(2+) signal response using aequorin-based Ca(2+) reporter system. STAR Protoc. 2021;2: 100558.
Keller JM, Frieboes MJ, Jodecke L, Kappel S, Wulff N, Rindfleisch T, Sandoval-Ibanez O, Gerlach I, Thiele W, Bock R, et al. Eukaryote-specific assembly factor DEAP2 mediates an early step of photosystem II assembly in Arabidopsis. Plant Physiol. 2023;193:1970–86.
Zhang Y, Li J, Gao C. Generation of stable transgenic rice (Oryza sativa L.) by Agrobacterium-mediated transformation. Curr Protoc Plant Biol. 2016;1:235–46.
Li J, Chen L, Liang J, Xu R, Jiang Y, Li Y, Ding J, Li M, Qin R, Wei P. Development of a highly efficient prime editor 2 system in plants. Genome Biol. 2022;23:161.
Zhang X, Henriques R, Lin SS, Niu QW, Chua NH. Agrobacterium-mediated transformation of Arabidopsis thaliana using the floral dip method. Nat Protoc. 2006;1:641–6.
Download references
Acknowledgements
We thank Qijun Chen from China Agricultural University for sharing the pHEE401E plasmid.
Review history
The review history is available as Additional file 3 .
Peer review information
Tim Sands was the primary editor of this article and managed its editorial process and peer review in collaboration with the rest of the editorial team.
This work was supported by the Taishan Scholar Foundation of Shandong Province (TSQN202103160 to Huawei Zhang), the Excellent Youth Foundation of Shandong Scientific Committee (ZR202103010168 to Huawei Zhang), and the National Key R&D Program of China (2022YFD1201700 to Lijing Liu).
Author information
Xiang Wang, Wenbo Pan, Chao Sun, Hong Yang, and Zhentao Cheng contributed equally to this work.
Authors and Affiliations
The Key Laboratory of Plant Development and Environmental Adaptation Biology, Ministry of Education, School of Life Sciences, Shandong University, Qingdao, Shandong, China
Xiang Wang, Fei Yan, Guojing Ma & Lijing Liu
National Key Laboratory of Wheat Improvement, Peking University Institute of Advanced Agricultural Sciences, Shandong Laboratory of Advanced Agriculture Sciences in Weifang, Weifang, Shandong, China
Wenbo Pan, Hong Yang, Zhentao Cheng, Yun Shang & Huawei Zhang
New Cornerstone Science Laboratory, Center for Genome Editing, Institute of Genetics and Developmental Biology, Chinese Academy of Sciences, Beijing, China
Chao Sun, Rui Zhang & Caixia Gao
College of Advanced Agricultural Sciences, University of Chinese Academy of Sciences, Beijing, China
Chao Sun & Caixia Gao
You can also search for this author in PubMed Google Scholar
Contributions
H.Z. and L.L. designed the experiments. X.W., W.P., C.S., H.Y., Z.C. F.Y., G.M., and Y. S. performed the experiments. X.W., W.P., C.S., and R.Z. analyzed the results. H.Z., L.L., and C.G. supervised the project and wrote the manuscript.
Corresponding authors
Correspondence to Caixia Gao , Lijing Liu or Huawei Zhang .
Ethics declarations
Ethics approval and consent to participate.
Not applicable.
Consent for publication
Competing interests.
The authors’ patent based on the results reported in this paper has been granted (patent number ZL202211484789.3) and does not prevent other from using the described method to screen.
Additional information
Publisher’s note.
Springer Nature remains neutral with regard to jurisdictional claims in published maps and institutional affiliations.
Supplementary Information
13059_2024_3358_moesm1_esm.docx.
Additional file 1: Fig. S1. Schematic overview of the pipeline for generating large scale mutant pool. Fig. S2. Schematic overview of targets used for checking the editing efficiency of pHEE901-A3A. Fig. S3. The mutated alleles generated by pHEE901-A3A at AG-T2, ARF2 and HAB1 target sites in the T1 generation. Fig. S4. The mutated alleles generated by pHEE901-A3A at AG and EPSPS sites in the T1 generation. Fig. S5. The mutated alleles generated by pHEE901-A3A at AG and EPSPS sites in the T2 generation. Fig. S6. The amino acids sequences at AG and EPSPS targets in the T1 and T2 generation mutants. Fig. S7. The DNA sequences encoding the effective mutated proteins at AG target in the T2 generation. Fig. S8. The alignment of ALSs from Arabidopsis and rice. Fig. S9. The numbers of T1 plants for each sgRNA used for ALS evolution. Fig. S10. Mutation forms at the DNA and protein levels at the ALS targets detected in herbicide-resistant plants. Fig. S11. No obvious growth penalty was observed in ALS mutants. Fig. S12. The herbicide resistant phenotypes of different ALS mutants. Fig. S13. No obvious growth penalty was observed in Os ALS genome edited rice mutants. Fig. S14. The herbicide resistant phenotypes of Os ALS genome edited rice mutants. Fig. S15. Sequence alignment of HPPDs from Arabidopsis and crops. Fig. S16. Mutation forms at the DNA and protein levels at the HPPD targets detected in herbicide-resistant plants. Fig. S17. Amino acid substitutions detected in herbicide resistant HPPD mutant forms. Fig. S18. The diagram and sequences used for the prime editing of ALS in rice.
13059_2024_3358_MOESM2_ESM.xlsx
Additional file 2: Table S1. The ratios of different mutation types at the five endogenous targets in the T1 generation. Table S2. The base editing efficiency at each nucleotide in the protospacers of five targets in T1 plants. Table S3. The ratios of different mutation types at AG and EPSPS sites in the T2 generation. Table S4. The genotypes and mutation forms of herbicide resistant plants screened out by the efficient germline-specific evolution system. Table S5. The primers used in this study.
Additional file 3. Peer review history.
Rights and permissions.
Open Access This article is licensed under a Creative Commons Attribution-NonCommercial-NoDerivatives 4.0 International License, which permits any non-commercial use, sharing, distribution and reproduction in any medium or format, as long as you give appropriate credit to the original author(s) and the source, provide a link to the Creative Commons licence, and indicate if you modified the licensed material. You do not have permission under this licence to share adapted material derived from this article or parts of it. The images or other third party material in this article are included in the article’s Creative Commons licence, unless indicated otherwise in a credit line to the material. If material is not included in the article’s Creative Commons licence and your intended use is not permitted by statutory regulation or exceeds the permitted use, you will need to obtain permission directly from the copyright holder. To view a copy of this licence, visit http://creativecommons.org/licenses/by-nc-nd/4.0/ .
Reprints and permissions
About this article
Cite this article.
Wang, X., Pan, W., Sun, C. et al. Creating large-scale genetic diversity in Arabidopsis via base editing-mediated deep artificial evolution. Genome Biol 25 , 215 (2024). https://doi.org/10.1186/s13059-024-03358-9
Download citation
Received : 01 March 2024
Accepted : 29 July 2024
Published : 09 August 2024
DOI : https://doi.org/10.1186/s13059-024-03358-9
Share this article
Anyone you share the following link with will be able to read this content:
Sorry, a shareable link is not currently available for this article.
Provided by the Springer Nature SharedIt content-sharing initiative
- Base editing
- Artificial evolution
- Germline-specific
- High-throughput screening
Genome Biology
ISSN: 1474-760X
- Submission enquiries: [email protected]
- General enquiries: [email protected]
This page has been archived and is no longer updated
Biodiversity and Ecosystem Stability

Introduction: Biodiversity, Stability, and Ecosystem Functioning
Climate change and other human-driven (anthropogenic) environmental changes will continue to cause biodiversity loss in the coming decades (Sala et al . 2000), in addition to the high rates of species extinctions already occurring worldwide (Stork 2010). Biodiversity is a term that can be used to describe biological diversity at a variety of different scales, but in this context we will focus on the description of species diversity. Species play essential roles in ecosystems, so local and global species losses could threaten the stability of the ecosystem services on which humans depend (McCann 2000). For example, plant species harness the energy of the sun to fix carbon through photosynthesis, and this essential biological process provides the base of the food chain for myriad animal consumers. At the ecosystem level, the total growth of all plant species is termed primary production, and — as we'll see in this article — communities composed of different numbers and combinations of plant species can have very different rates of primary production. This fundamental metric of ecosystem function has relevance for global food supply and for rates of climate change because primary production reflects the rate at which carbon dioxide (a greenhouse gas) is removed from the atmosphere. There is currently great concern about the stability of both natural and human-managed ecosystems, particularly given the myriad global changes already occurring. Stability can be defined in several ways, but the most intuitive definition of a stable system is one having low variability (i.e., little deviation from its average state) despite shifting environmental conditions. This is often termed the resistance of a system. Resilience is a somewhat different aspect of stability indicating the ability of an ecosystem to return to its original state following a disturbance or other perturbation.
Species Identity, Functional traits, and Resource-Use
Species diversity has two primary components: species richness (the number of species in a local community) and species composition (the identity of the species present in a community). While most research on the relationship between ecosystem diversity and stability has focused on species richness, it is variation in species composition that provides the mechanistic basis to explain the relationship between species richness and ecosystem functioning. Species differ from one another in their resource use, environmental tolerances, and interactions with other species, such that species composition has a major influence on ecosystem functioning and stability.
The traits that characterize the ecological function of a species are termed functional traits, and species that share similar suites of traits are often categorized together into functional groups. When species from different functional groups occur together, they can exhibit complementary resource-use, meaning that they use different resources or use the same resources at different times. For example, two animal predators may consume different prey items, so they are less likely to compete with one another, allowing higher total biomass of predators in the system. In the case of plants, all species may utilize the same suite of resources (space, light, water, soil nutrients, etc.) but at different times during the growing season — for example, early- and late-season grasses in prairies. Increasing species diversity can influence ecosystem functions — such as productivity — by increasing the likelihood that species will use complementary resources and can also increase the likelihood that a particularly productive or efficient species is present in the community. For example, high plant diversity can lead to increased ecosystem productivity by more completely, and/or efficiently, exploiting soil resources (e.g., nutrients, water). While primary production is the ecosystem function most referred to in this article, other ecosystem functions, such as decomposition and nutrient turnover, are also influenced by species diversity and particular species traits.
Diversity-Stability Theory
Theoretical models suggest that there could be multiple relationships between diversity and stability, depending on how we define stability (reviewed by Ives & Carpenter 2007). Stability can be defined at the ecosystem level — for example, a rancher might be interested in the ability of a grassland ecosystem to maintain primary production for cattle forage across several years that may vary in their average temperature and precipitation. Figure 1 shows how having multiple species present in a plant community can stabilize ecosystem processes if species vary in their responses to environmental fluctuations such that an increased abundance of one species can compensate for the decreased abundance of another. Biologically diverse communities are also more likely to contain species that confer resilience to that ecosystem because as a community accumulates species, there is a higher chance of any one of them having traits that enable them to adapt to a changing environment. Such species could buffer the system against the loss of other species. Scientists have proposed the insurance hypothesis to explain this phenomenon (Yachi & Loreau 1999). In this situation, species identity — and particular species traits — are the driving force stabilizing the system rather than species richness per se (see Figure 2).

In contrast, if stability is defined at the species level, then more diverse assemblages can actually have lower species-level stability. This is because there is a limit to the number of individuals that can be packed into a particular community, such that as the number of species in the community goes up, the average population sizes of the species in the community goes down. For example, in Figure 2, each of the simple communities can only contain three individuals, so as the number of species in the community goes up, the probability of having a large number of individuals of any given species goes down. The smaller the population size of a particular species, the more likely it is to go extinct locally, due to random — stochastic — fluctuations, so at higher species richness levels there should be a greater risk of local extinctions. Thus, if stability is defined in terms of maintaining specific populations or species in a community, then increasing diversity in randomly assembled communities should confer a greater chance of destabilizing the system.
Experiments and Observations Can Evaluate the Diversity-Stability Relationship
A wealth of research into the relationships among diversity, stability, and ecosystem functioning has been conducted in recent years (reviewed by Balvanera et al . 2006, Hooper et al . 2005). The first experiments to measure the relationship between diversity and stability manipulated diversity in aquatic microcosms — miniature experimental ecosystems — containing four or more trophic levels, including primary producers, primary and secondary consumers, and decomposers (McGrady-Steed et al . 1997, Naeem & Li 1997). These experiments found that species diversity conferred spatial and temporal stability on several ecosystem functions. Stability was conferred by species richness, both within and among functional groups (Wardle et al . 2000). When there is more than one species with a similar ecological role in a system, they are sometimes considered "functionally redundant." But these experiments show that having functionally redundant species may play an important role in ensuring ecosystem stability when individual species are lost due to environmental changes, such as climate change.
More recently, scientists have examined the importance of plant diversity for ecosystem stability in terrestrial ecosystems, especially grasslands where the dominant vegetation lies low to the ground and is easy to manipulate experimentally. In 1995, David Tilman and colleagues established 168 experimental plots in the Cedar Creek Ecosystem Science Reserve, each 9 x 9 m in size (Figure 3A), and seeded them with 1, 2, 4, 8 or 16 species drawn randomly from a pool of 18 possible perennial plant species (Tilman et al . 2006). Plots were weeded to prevent new species invasion and ecosystem stability was measured as the stability of primary production over time. Over the ten years that data were collected, there was significant interannual variation in climate, and the researchers found that more diverse plots had more stable production over time (Figure 3B). In contrast, population stability declined in more diverse plots (Figure 3C). These experimental findings are consistent with the theory described in the prior section, predicting that increasing species diversity would be positively correlated with increasing stability at the ecosystem-level and negatively correlated with species-level stability due to declining population sizes of individual species.
Experiments manipulating diversity have been criticized because of their small spatial and short time scales, so what happens in naturally assembled communities at larger spatial scales over longer time scales? In a 24-year study of naturally assembled Inner Mongolia grassland vegetation, Bai et al . (2004) observed variation in the biomass of species, functional groups, and the whole community in response to strong interannual variation in growing-season precipitation. They found that while the abundance of individual species fluctuated, species within particular functional groups tended to respond differently such that a decrease in the abundance of one species was compensated for by an increase in the abundance of another. This compensation stabilized the biomass productivity of the whole community in a fluctuating environment (see Figure 1). These findings demonstrate that local species richness — both within and among functional groups — confers stability on ecosystem processes in naturally assembled communities.
Experiments in aquatic ecosystems have also shown that large-scale processes play a significant role in stabilizing ecosystems. A whole-lake acidification experiment in Canada found that although species diversity declined as a result of acidification, species composition changed significantly and ecosystem function was maintained (Schindler 1990). This suggests that given sufficient time and appropriate dispersal mechanisms, new species can colonize communities from the regional species pool and compensate for those species that are locally lost (Fischer et al . 2001). This observation emphasizes the importance of maintaining connectivity among natural habitats as they experience environmental changes.
Evidence from multiple ecosystems at a variety of temporal and spatial scales, suggests that biological diversity acts to stabilize ecosystem functioning in the face of environmental fluctuation. Variation among species in their response to such fluctuation is an essential requirement for ecosystem stability, as is the presence of species that can compensate for the function of species that are lost. While much of the evidence presented here has focused on the consequences of changes in species diversity on primary production in natural ecosystems, recent research has found similar relationships between species diversity and ecosystem productivity in human-managed ecosystems (e.g., Jactel et al . 2005).
References and Recommended Reading
Bai, Y. et al. Ecosystem stability and compensatory effects in the Inner Mongolia grassland. Nature 431 , 181–184 (2004).
Balvanera, P. et al. Quantifying the evidence for biodiversity effects on ecosystem functioning and services. Ecology Letters 9 , 1146–1156 (2006).
Fischer J. M. et al . Compensatory dynamics in zooplankton community responses to acidification: Measurement and mechanisms. Ecological Applications 11 , 1060–1072 (2001).
Hooper, D. U. et al. Effects of biodiversity on ecosystem functioning: A consensus of current knowledge and needs for future research. Ecological Monographs 75 , 3–35 (2005).
Ives, A. R. & Carpenter, S. R. Stability and diversity of ecosystems. Science 317 , 58–62 (2007).
Jactel, H. et al. A test of the biodiversity-stability theory: Meta-analysis of tree species diversity effects on insect pest infestations, and re-examination of responsible factors. Forest Diversity and Function 176 , 235–262 (2005).
McCann, K. S. The diversity-stability debate. Nature 405 , 228–233 (2000).
McGrady-Steed, J. et al. Biodiversity regulates ecosystem predictability. Nature 390 , 162–165 (1997).
Naeem, S. & Li, S. Biodiversity enhances ecosystem reliability. Nature 390 , 507–509 (1997).
Naeem, S. et al . Declining biodiversity can alter the performance of ecosystems. Nature 368 , 734–737 (1994).
Sala, O. E. et al. Global biodiversity scenarios for the year 2100. Science 287 , 1770–1774 (2000).
Schindler, D. W. Experimental perturbations of whole lakes as tests of hypotheses concerning ecosystem structure and function. Oikos 57 , 25–41 (1990).
Stork, N. E. Re-assessing current extinction rates. Biodiversity and Conservation 19 , 357–371 (2010).
Tilman, D. et al. Biodiversity and ecosystem stability in a decade-long grassland experiment. Nature 441 , 629–632 (2006).
Wardle, D. A. et al. Stability of ecosystem properties in response to above-ground functional group richness and composition. Oikos 89 , 11–23 (2000).
Article History
Flag inappropriate.


Email your Friend

- | Lead Editor:

Within this Subject (24)
- Basic (13)
- Intermediate (5)
- Advanced (6)
Other Topic Rooms
- Ecosystem Ecology
- Physiological Ecology
- Population Ecology
- Community Ecology
- Global and Regional Ecology
- Conservation and Restoration
- Animal Behavior
- Teach Ecology
- Earth's Climate: Past, Present, and Future
- Terrestrial Geosystems
- Marine Geosystems
- Scientific Underpinnings
- Paleontology and Primate Evolution
- Human Fossil Record
- The Living Primates

© 2014 Nature Education
- Press Room |
- Terms of Use |
- Privacy Notice |

Visual Browse
/cdn.vox-cdn.com/uploads/chorus_image/image/73504432/Eater_Pizza_Joe_sPizza_005.0.jpeg)
Filed under:
No Taste Like Home
New york pizza is an evolution in four acts.
The city with the highest diversity of styles, a hundred years in the making
Share this story
- Share this on Facebook
- Share this on Twitter
- Share All sharing options
Share All sharing options for: New York Pizza Is an Evolution in Four Acts
Call me biased, but New York is the most dynamic city in the world when it comes to pizza. Waves of Italian immigrants, our city’s hustle culture, updates in oven technology, and the influence of the cheffy era have led to the evolution and diversification of one of the city’s most famous meals.
New York-style pizza is one of the city’s few iconic foods that many of us judge in relation to what we ate in our childhood — critical faculties out the window. Some of us go for a thin sheen of sweet tomatoes and a blanket of mozzarella. Others insist on a savory tomato, flecked with Italian oregano and a smattering of (browned) cheese. One contingent likes a chewy crust that’s firm enough to fold. Another looks for a crust that’s leopard-spotted at the bottom — the list goes on.

Pizza in New York is so iconic that, similar to Italians and their staunch food rules by region , aficionados have attempted to establish a set of unwritten rules as to what makes “the best” — a little unusual in a city, not to mention country, that prizes innovation, especially when it comes to food. And yet, “the rules” are admittedly flexible, based on the age of the rule-maker, where they’d grown up, and their favorite pizzerias.
As the case has been in the famous pizza city of Naples — where the Lombardi’s founders are from — pizza has evolved from a blue-collar food to one that’s enjoyed by people across socioeconomic backgrounds, notes Marlena Spieler, in her book, A Taste of Naples: Neapolitan Culture, Cuisine, and Cooking.
Though pizza was around before it came to New York, let’s say for our purposes that New York-style pizza is its own thing that entered the city’s food lexicon well over 100 years ago. Since then, pizza-makers all around the city have been tweaking recipes, changing up ovens, and swapping in new ingredients. The result? While New York pizza — and its offspring, the New York slice — is always recognizable, an old-school versus a new-school pie is, for pizza fanatics, like comparing apples to oranges.
With the help of some of the city’s most knowledgeable pizza folks, we’re laying out a celebration of four stages in the evolution of New York pizza — all of which are still available at shops around the city.
The coal-oven pie started it all
The coal-oven pie is a precursor of the New York slice, with origins, according to lore , at Lombardi’s on Spring Street back in the early 1900s, perhaps earlier . It’s the locus for a style that, due to super high heat, says Daniel Young, author of Where to Eat Pizza: The Last Word on the Slice, sports a smokiness, along with a drier, leopard-charred crust on the undercarriage and the cornicione — or as critic Robert Sietsema and the late Jonathan Gold call it, “the bone.” In short, coal-fired pizza establishes the baseline.
Scott Wiener, of Scott’s Pizza Tours and the founder of Slice Out Hunger , has his own observations about the classic New York style: The dough — flour, salt, water, and yeast — is perhaps the most dense of the evolutions of New York pizzas we’re talking about here. As for toppings, it’s “simple, unseasoned tomato and mozzarella, and that’s it,” he says.
Places that show off this older style include Arturo’s on West Houston, Patsy’s in East Harlem, and John’s of Bleecker Street . Around since 1929, from Giovanni “John” Sasso, John’s first opened on Greenwich and moved to 278 Bleecker Street, at Jones Street. Sasso ran his business until he sold the place to the Vesce Brothers in 1954, according to the website. Augustine “Chubby” Vesce bought the business from his brothers, and continued to own and operate John’s of Bleecker Street until he died in 1984. Today, it’s owned by Peter Castellotti Jr. and Robert Vittoria.
When visiting John’s , you still have to order a whole pie. Graffiti-carved walls frame the interior, while the booths have recently been replaced. John’s still offers a throwback-style, 14-inch or 16-inch “John’s Classic” pizza in the original with tomato and mozzarella; the Sasso, with Parmesan, oregano, and black pepper; or the margherita, with fresh basil. Want a glass of Chianti with that? Order one poured nearly to the rim and feast in a booth.
John’s of Bleecker Street and its pizza.
Bakers Pride ovens change the game
The invention of the gas deck-oven — ovens like Bakers Pride , which came out of the Bronx in the mid-1940s — is essential to the evolution of the New York pizza. This is when we started seeing the proliferation of pizza sold by the slice.
“[The slice] is flexible. It’s portable. And it’s an icon for New York becoming a famous pizza city,” says Wiener of pizza in this era. He describes the style as “crispy and chewy at the same time,” one-eighth of a large, sharable pie, with each slice bigger than the paper plate it’s served on. “Reheating is built into the style,” he says, to which Carlo Mirarchi, co-founder of Roberta’s in Bushwick, confirms.
“The evolution of New York-style pizza is really about technology,” says Mirarchi. This next-level pizza is cooked at a lower temperature, say 500 to 700 degrees, as opposed to 900 degrees or more for coal-fired pizza. From the 1950s through the 1970s, the slice grew in popularity beyond the earlier coal-fired era.
Joe’s Pizza (7 Carmine Street, at Sixth Avenue) from Joe Pozzuoli open since 1975 and also in the Village, is “the quintessential New York slice... big, floppy, foldable, [that] glistens with a sheen of oil,” writes Wiener on his site . There are a million examples of this style, including places like John’s Pizzeria in Elmhurst since 1960; Luigi’s in Park Slope since 1973, and more.
Outside Joe’s, and its pizza.
Opening the floodgates in the wood-fired era
In the late 1990s through the aughts, two evolutions pushed people’s expectations for pizza. One is New York’s wood-fired Neapolitan pie, ushered in by pizza-makers like Anthony Mangieri, who had his own rules for his shop, including no substitutions. Mangieri has opened several iterations of his lone pizzeria, but it started as Una Pizza Napoletana , first in the 1990s in New Jersey, later in New York in 2004, after which it moved to San Francisco, then back to New York, where it remains today . Other pizza shops adhere more strictly to the VPN rules for making Neapolitan pizzas, established in Italy in 1984 (00 or single-O flour, max to 35 cm diameter, San Marzano or similar plum tomatoes, sparse toppings), such as Michael Ayoub, who opened Fornino in 2004. Roberto Caporuscio, a Neapolitan evangelist, opened Kesté in New York in 2009. His daughter Giorgia Caporuscio carries the torch and is this year’s pizzaiolo of the year from Italy’s 50 Top Pizza for her pizza at Don Antonio.
With the rise of wood-fired Neapolitan pizzas, known for soft crust and fior di latte or buffalo mozzarella (less industrial cheese than what is often used for gas-oven pizzas), New York customers were guided back toward the whole-pie style of ordering. In addition, they had to get used to spending a bit more on pizza, were groomed to seek out higher-quality toppings, and began to expect wines by the glass that were more interesting than, say, the house red.
But it was Roberta’s (261 Moore Street, at Bogart Street), which opened in Bushwick in 2008, that made pizza a lifestyle and a fashion, notes Wiener. While Roberta’s embraced the of-the-moment, wood-fired baking, it tossed away rules when it came to ingredients.
“Roberta’s is a gateway to what we accept for pizza: how they’re topped and what we’ll spend,” says Wiener. The crust is charred; like the Neapolitan style, they’re using “good tomatoes,” perhaps lightly salted. It’s at this moment, notes Wiener, when it becomes acceptable for each person at a table to eat their own pie.
Yet the Roberta’s founder noted that the choice in style was shaped by wanting to bring back the communal nature of eating pizza — like at Grimaldi’s and Patsy’s — that is less of a priority in a typical New York slice shop. “We wanted to have sit-down pizzas in the middle of the table, offering slices that everyone could grab,” he says.
In a review titled “Artisanal-Everything Roberta’s Defies the Stereotypes,” former Eater critic Ryan Sutton notes Roberta’s transformation from “a pizza parlor” into “one of New York’s most quintessential kitchens,” as much for the pizza as the rest of the menu. Roberta’s is a style he defines as “a stunning New York-Neapolitan hybrid, without that soupy center,” where, even with novel toppings combinations, “The margherita pizza tastes like it should — a tightrope balance of tangy tomatoes, mozzarella, and basil.”
Roberta’s continues to pioneer New York pizza styles, even as others innovate beyond them. Earlier this year, Roberta’s segued to the world of a slice shop, opening R Slice , next door to its Bushwick flagship, where it’s using the same dough to turn out something new for the brand. Here, it’s the Bakers Pride-style slice, with new combinations like the Bee Sting with honey, and the Fire and Ice (stracciatella with ’nduja). And just last month , the brand opened its first location in Manhattan, a two-in-one R Slice and Roberta’s in the Penn District at Seventh Avenue and 33rd Street.
Outside R Slice, now open in Manhattan, and its pizza.
The dawn of new-wave pizza
This brings us to our current new-wave era: not entirely driven by wild ingredient combinations or bulbous balls of burrata on pies — though there’s that, too. Pizza has diversified, with New Yorkers wanting more styles, more surprise toppings, more cultural mash-ups, and more attention to the crust-as-good-as-fancy-bread.
A bakery movement has helped fuel current tastes, with customers better understanding that more than half of what makes for good pizza is in the flour, the fermentation style, and hydration of the dough, much like bread. The type of oven makes a difference, too, whether it’s made in the increasingly popular electric ovens, gas ovens, or wood-fired ovens (with coal ovens on the way to extinction).
With the opening of places around 2015 like L’Industrie in Brooklyn and Scarr’s (35 Orchard Street, at Hester Street) from Scarr Pimentel — with its in-house milled flour — people’s expectations for New York pizza have leveled up.
This era is also intentional with ingredients, says Wiener. “What’s interesting is that new-wave pizza has trickled down to old-school slice shops,” he says, that are in turn diversifying their offerings. Wiener notes places like Mama’s Too (that Pete Wells in the Times called “a genetically modified pizza”) and the ’90s-themed Upside Pizza as examples of these pies.
Even those updating the pizza of their childhood is considered new wave, with upgraded ingredients and newer digs. One example is Chrissy’s Pizza , the late-night, reservation-only whole pie destination in the back of Superiority Burger in the East Village (with a soon-to-open standalone location). It’s one of the most challenging pizzas to get in the city, made by Chris Hansell, who used to sell a set number of pizzas a night out of his Bushwick apartment, starting in 2022 . His pizzas, made with “good tomatoes,” oregano, pecorino Romano before the bake, and Grana Padano on the finish, offer a crisp crust for a well-done slice that slightly droops at the tip.
“My inspiration for pizzas are the places my father took me,” he says, citing a few pizzerias in Queens as well as where he grew up in Valley Stream on Long Island. “There’s a certain flavor that it used to taste like that’s a little different from what slice shops serve now.”
Outside Scarr’s, and its pizza.

‘Criminal Minds: Evolution’ Didn’t Do Enough With the Gold Star Program

Your changes have been saved
Email is sent
Email has already been sent
Please verify your email address.
You’ve reached your account maximum for followed topics.
Editor's Note: The following contains spoilers for the Season 2 finale of Criminal Minds: Evolution.
The Big Picture
- In Criminal Minds , the BAU uses a profiling technique that comes from the study of epigenetics.
- Criminal Minds: Evolution Season 2 focused more on hunting criminals than delving into their psyche.
- Epigenetics introduced in the show offered potential but lacked in-depth exploration into killers' past and psychology.
What makes Criminal Minds stand out from other police procedurals is its in-depth look into the twisted mind of a serial killer, from the abuse they suffered in their childhood to the compulsions they face in the present. The BAU's profiling techniques stem from the field of psychological epigenetics, which studies how environmental factors interact with genetics . In Criminal Minds ' case, this can frequently be seen as childhood abuse or stressors being the environmental factors which trigger the criminals' genetic predisposition to homicidal tendencies — namely in psychopaths. Criminal Minds: Evolution Season 2 finally uttered the word epigenetics, and though the series is technically based on the concept, it was satisfying and exciting to see the term finally be explored.
The entirety of the recent season revolved around the Gold Star case, which was a dark program that exploited the principles of epigenetics to groom psychopathy in selected children. The mere mention of the science opened up new possibilities of how the show could investigate serial killers. However, the season ended up being consumed by "the hunt," wasting a valuable opportunity to delve into the unique relationship between genetics and homicide . Considering what makes Criminal Minds truly special is its psychological analysis of these criminals , it is particularly disappointing that adrenaline triumphed over true psychological investigation.
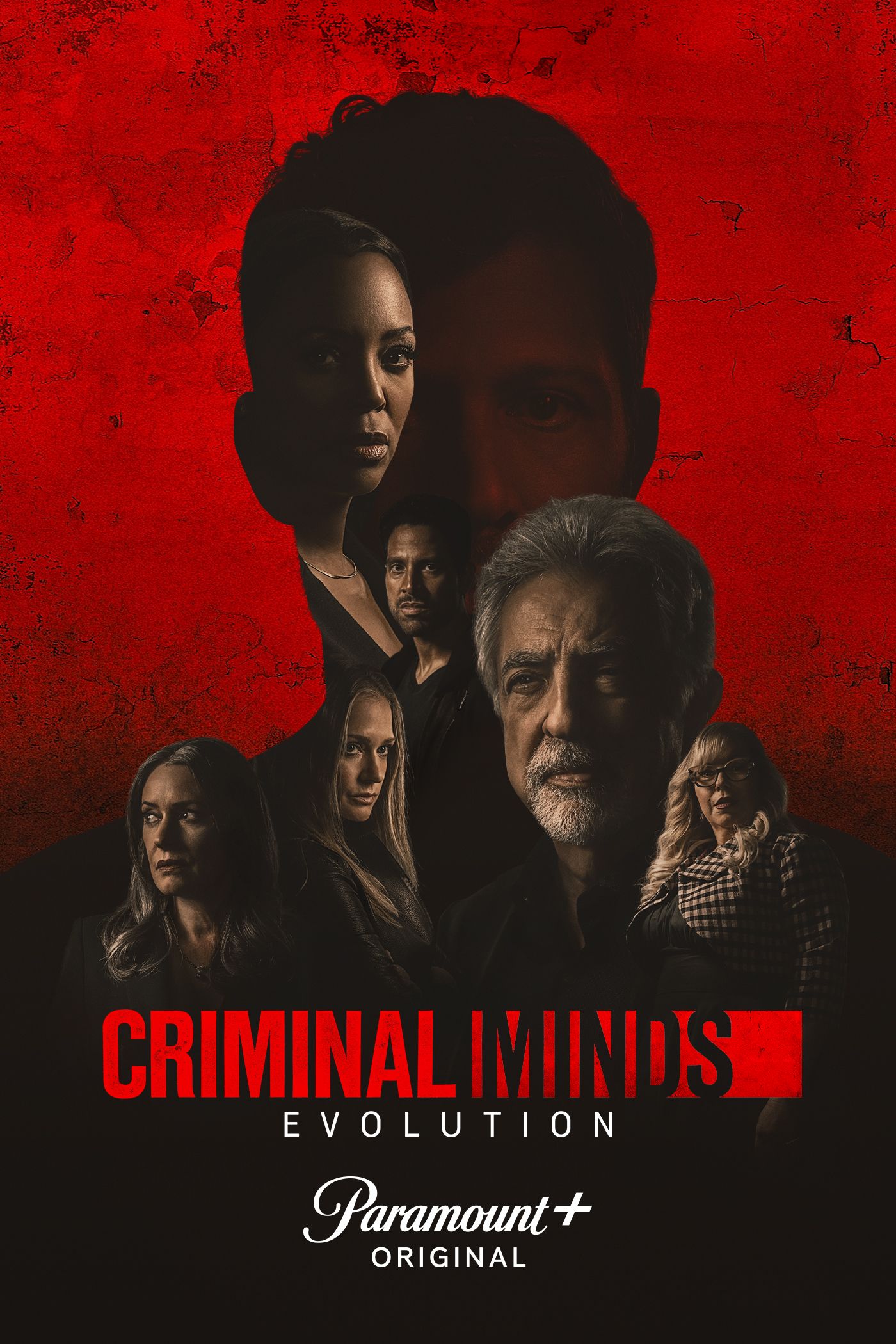
Criminal Minds
Criminal Minds follows the elite team of FBI profilers in the Behavioral Analysis Unit (BAU) as they analyze and track down the country’s most dangerous serial killers and criminals. Led by experienced agents like Jason Gideon, Aaron Hotchner, and later David Rossi, the team delves into the minds of the perpetrators, using psychological profiling and investigative techniques to anticipate their next moves. Each episode features intense and often chilling cases, while also exploring the personal lives and challenges faced by the team members.
'Criminal Minds: Evolution' Season 2 Focused on the Wrong Story
In lieu of focusing on one case per episode, Evolution Season 2 really delves into one overarching case throughout the whole season, with only a couple of standalone cases being littered in. We were introduced to Gold Star at the end of Season 1, where Elias Voit ( Zach Gilford ) innocuously uttered the term that would later haunt the BAU. The team discovered that the Gold Star program was used to cultivate psychopathy in adolescents and children who were already predisposed to it . Throughout the season, they chase down five members of Gold Star who had grown up in the Stuart house, but when the program ceased, they were taken in by Frank Church ( Tuc Watkins ) who molded the psychopaths into fully-fledged assassins. He had falsely promised that he was taking down institutions like the Stuart House, but when his lies were unraveled, he was forced to hire mercenaries to hunt the team down. However, the Gold Star killers wrought their own vengeance instead, and as such, the BAU was able to start tracking them down, namely Jade ( Liana Liberato ) and Damien ( David Garelik ).
With the entire season revolving around Gold Star, it would have become the perfect opportunity to hone into their psyche and their past. Criminal Minds managed to successfully do this in Elias' prolonged storyline , giving us an unsettling insight into the jarring mechanisms that make him a psychopath and the side that still loved his family. But with Jade and Damien, the show primarily reveled in the hunt, only offering a brief snapshot of what their tumultuous psychology was really like, mainly through Liberato and Garelik's tortured performances when their characters get caught. While the profiling techniques used to determine Jade and Damien's next moves are a thrilling, race-against-the-clock, adrenaline rush, the exploration into how they transformed into killers is incomplete, especially considering the amount of time spent on them. New characters, foreshadowed revelations, and fitting Elias into the premise are all exciting prospects, but they shouldn't replace the psychological aspect that is the essence of Criminal Minds .
Epigenetics Is Introduced and Forgotten in 'Criminal Minds: Evolution'
When epigenetics was introduced alongside Jill Gideon ( Felicity Huffman ) , it broadened how Criminal Minds could portray its psychoanalytical tools by linking it to genetics. It is only in Episode 8 that we see echoes of how promising epigenetics could be, where Jill and David Rossi (Joe Mantegna) sit together and discuss potential candidates for the Gold Star program using biographies . They read about children who experienced neglect and abuse and also demonstrated a tendency towards psychopathy through behaviors like setting fires and torturing animals. Traditionally in the show, we see this called the "homicidal triad," and as such, it is rewarding to learn how it is connected to real science rather than theory. Jill exhibits the physical application of this by deconstructing Damien's beliefs in conspiracy theories. However, the scene feels incomplete, since we only briefly see how these beliefs were instilled in him in the first place.
We just have a vague sense of the Stuart house creating the right environment to groom psychopaths, with the only examples being sexual assault and electric shocks – there was no other real insight into what occurred there . When Criminal Minds explored the psyche of Elias throughout a season, it delivered flashbacks of his relationship with his uncle and his childhood, explaining a great deal of his behavior. Jade and Damien's flashbacks were extremely limited in comparison, especially considering the extent of their grooming . While torture is an element to ingraining conspiracy theories into them, gaining insight into their schedule, the living conditions, and the actual brainwashing at both the Stuart house and Church's compound would have fleshed out their psychologies and their characters immensely.
The idea of linking epigenetics to conspiracy theories is also an intriguing idea that was underdeveloped in the show. Touching on what sort of qualities made them more susceptible to brainwashing would have benefited the show's exploration into how they interact. The only explanation offered was that Church preyed on the group's need for vengeance on the Stuart house, mixed with wanting to save other children from their fate. However, with no real insight into the trauma they endured in the Stuart house, it all feels fairly vague and arbitrary . Truly, the only issue here is that the show reserved more time for the BAU hunting them down, rather than allowing us to fully understand them.
The White Papers' Scandal Is Underplayed in 'Criminal Minds: Evolution'
Another aspect of epigenetics that Criminal Minds vastly underplays is the white papers. Written by Rossi, Jason Gideon ( Mandy Patinkin ) and Jill, the papers were a theoretical thought experiment on what the perfect conditions would be to nurture a child's predisposition to psychopathy . While Rossi and Jason tried to bury it after recognizing the potential consequences of its release, Jill used it to establish the Stuart house and many others like it -- a program that would curb growing psychopathy in children . However, she soon discarded the idea, not realizing that the experiment had continued without her knowledge and had grown into something more sinister.
While the FBI director points out the catastrophic impact it would make on the BAU's already precarious reputation, the team only glosses over the concern of being the root cause of this program — or, as Elias puts it, the North Star. Delving into the BAU's accountability for the white papers would have been a fascinating development for Rossi's character . The profiler is already at the forefront of Evolution as his mental state deteriorates with Elias' presence, but casting light on his writing ventures and its potential impact would have been a compelling arc.
The show already touches on this through Elias, who hints that he learned to hide his psychopathy by perusing Rossi's books . And now, the Gold Star program creates a more concrete link between the BAU's work and the growing sophistication of serial killers. It almost becomes a meta-commentary on how police procedurals, like Criminal Minds , could potentially impact real-life crime . But like everything related to epigenetics, it loses valuable screen time and gets lost under the folds of the hunt. Though the season was fresh in its approach to dissecting criminal minds, a more insightful look into the intriguing concepts it introduced would have given the season the analytical flavor we love so much.
Criminal Minds: Evolution is available to stream now on Paramount+ in the U.S.
WATCH ON PARAMOUNT+
- TV Features

- Undergraduate Admission
- Graduate Admission
- Tuition & Financial Aid
- Communications
- Health Sciences and Human Performance
- Humanities and Sciences
- Music, Theatre, and Dance
- IC Resources
- Office of the President
- Ithaca College at a Glance
- Awards and Accolades
- Five-Year Strategic Plan
- Fall Orientation
- Directories
- Course Catalog
- Undergraduate
STUDY ABROAD SCHOLARSHIP: Fund for Education Abroad - Spring 2025 applications due Sept. 18!

FUND FOR EDUCATION ABROAD OVERVIEW The Fund for Education Abroad (FEA) was established in 2010 as a 501(c)3 non-profit organization to enable more American college students to study abroad. Applicants from groups underrepresented in study abroad are given preference, in an effort to make the demographics of U.S. undergraduates studying abroad reflect the rich diversity of the U.S. population. Since 2010, FEA has awarded over $3.7 million in scholarships to 1186 undergraduates, helping students from all over the United States follow their dreams of studying abroad. Multiple scholarships are available through FEA, and just one application enables students to be considered for every scholarship for which they meet the criteria. Those scholarships include, in addition to the FEA General Scholarship, a range of special named & dedicated scholarships.
AWARD ELIGIBILITY FEA invests in deserving U.S. undergraduates who are least likely to study abroad. They consider financial need, demographic factors, and academic plan and preparedness to decide their scholarship recipients.
- Financial need is determined by the FEA Financial Aid Form that will be provided when you begin an application. Students must have a completed FAFSA for the current academic year.
- Demographic factors that are considered include first-generation college student status, racial identity, and community college experience.
- Academic plan and preparedness are determined by your essays and unofficial transcript.
To be eligible for an FEA scholarship, you must be a U.S. citizen, permanent resident, or DACA recipient; be currently enrolled as an undergraduate at a college or university in the US; receive credit at your home institution for the study abroad program; and have demonstrated financial need. APPLICATION DEADLINES The application deadline is September 18, 2024 for Spring 2025 programs. For Summer 2025, Fall 2025, and Academic Year 2025-26 programs, the application cycle will open in November 2024.
SPRING 2025 APPLICANT WEBINAR Watch the FEA Spring 2025 applicant webinar for information about eligibility requirements, application components, recent changes to the application form, and more: Fund for Education Abroad Spring 2025 Applicant Webinar on Vimeo
FOR MORE INFORMATION FEA Mission and Vision Additional Scholarships through FEA’s Access Partners Eligibility Application Instructions FAQs NEED MORE INFORMATION TO PLAN YOUR STUDY ABROAD EXPERIENCE? Schedule an appointment with a study abroad adviser - we'll help you find the program that's right for you. Please note: we recommend meeting with an academic adviser to discuss your study abroad needs and timeline BEFORE scheduling a one-on-one study abroad appointment.
Get notified of upcoming study abroad events, information and announcements: join the Study Abroad page on IC Engage , and follow us on Instagram and Facebook .
International Programs 074 Peggy Ryan Williams Center (607) 274-3306 [email protected]

COMMENTS
Biodiversity has a key role in maintaining healthy ecosystems and thereby sustaining ecosystem services to the ever-growing human population. To get an idea of the range of ecosystem services that we use daily, think of how much energy and time it would cost to make Mars (or some other Earth-like planet) hospitable for human life, for example, in terms of atmosphere regulation, freshwater ...
Eco-evolutionary dynamics are essential in shaping the biological response of communities to ongoing climate change. Here we develop a spatially explicit eco-evolutionary framework which features ...
Human beings get so many benefits from biodiversity. These services may include food, wood, nitrogen fixation, pollination, and beauty. Other services include maintenance of climate and life, prevention of overflow of water and famine, natural bug's control, and even spiritual enrichment.
The immediate applications of ecology and evolution research would be obvious to most, as would be the importance and inherent interest of more basic topics such as the evolution of organismal ...
The diversity of living forms and the unity of evolutionary processes are themes that have permeated the research and writing of Ernst Mayr, a Grand Master of evolutionary biology. The essays collected here are among his most valuable and durable: contributions that form the basis for much of the contemporary understanding of evolutionary biology.
Conclusion. Evolutionary perspectives provide invaluable insights for conservation biologists working to preserve biodiversity in a changing world. By considering the adaptive potential, evolutionary history and coevolutionary relationships of species, conservation efforts can be more targeted, effective and sustainable.
Concerns over the consequences of biodiversity loss for human well-being triggered the growth of biodiversity-ecosystem functioning (BEF) research, an important field of ecology over the past 25 ...
Evolution and Biodiversity: The evolutionary basis of biodiversity and its potential for adaptation to global change Report of an electronic conference, March 2010 ... In her essay on the genetic basis of evolution, she focused on two observations: Firstly, the variation between species was driven
It is critical, however, that an evolutionary perspective be incorporated into conservation planning (Hendry et al. 2010). 1. Evolution generates and maintains biodiversity and ecosystems Biodiversity is a product of evolution and, in the broadest sense, includes all biological variation on Earth, present and past.
Decreasing Biodiversity. In the past hundred years, biodiversity around the world has decreased dramatically. Thousands of species are in danger of extinction. Extinction is a natural process; some species naturally die out while new species evolve. But human activity has changed the natural processes of extinction and evolution.
Charles Darwin was a British naturalist who proposed the theory of biological evolution by natural selection. Darwin defined evolution as "descent with modification," the idea that species change over time, give rise to new species, and share a common ancestor. The mechanism that Darwin proposed for evolution is natural selection.
Two new studies, both in this issue of Current Biology, add to the growing body of work aiming to better understand anthropogenic changes in biodiversity ( Figure 1) by relating plant species extinctions and increases in species richness to rapid changes in climate and human use of land 10, 11. Figure 1. Published work relating to Anthropocene ...
500+ Words Essay on Biodiversity. Essay on Biodiversity - Biodiversity is the presence of different species of plants and animals on the earth. Moreover, it is also called biological diversity as it is related to the variety of species of flora and fauna. Biodiversity plays a major role in maintaining the balance of the earth.
Evolution and diversity result from the interactions between organisms and their environments and the consequences of these interactions over long periods of time. Organisms continually adapt to their environments, and the diversity of environments that exists promotes a diversity of organisms adapted to them. In recent years, new techniques and approaches have opened exciting new avenues of ...
Direction and Disruptive selection are needed for natural selection and to help with population diversity and evolution along with survival; without either of them, nothing would have been able to evolve and survive through the eons. ... evolution and diversity of life essay. Course: Introduction to Biology (SCI120) 79 Documents. Students ...
Biodiversity is measured in terms of attributes that explore the quality of nature; richness and evenness of the living organisms within an ecological niche. Biodiversity, Its Importance and Benefits. Apart from that, the paper is going to speculate on the most and least diverse species in the local area.
Biodiversity is essential for the processes that support all life on Earth, including humans. Without a wide range of animals, plants and microorganisms, we cannot have the healthy ecosystems that we rely on to provide us with the air we breathe and the food we eat. And people also value nature of itself.
Biodiversity is important to most aspects of our lives. We value biodiversity for many reasons, some utilitarian, some intrinsic. This means we value biodiversity both for what it provides to humans, and for the value it has in its own right. Utilitarian values include the many basic needs humans obtain from biodiversity such as food, fuel ...
The Biodiversity Heritage Library works collaboratively to make biodiversity literature openly available to the world as part of a global biodiversity community. ... Evolution and the Diversity of Life: Selected Essays by Ernst Mayr ... Title. Evolution and the Diversity of Life: Selected Essays by Ernst Mayr. By. Raikow, Robert J. Type ...
The 2019 United Nations Global assessment report on biodiversity and ecosystem services estimated that approximately 1 million species are at risk of extinction. This primarily human-driven loss ...
Evolution and the diversity of life : selected essays Bookreader Item Preview ... Evolution and the diversity of life : selected essays by Mayr, Ernst, 1904-Publication date 1976 Topics Evolution, Species Publisher Cambridge, Mass. : Belknap Press of Harvard University press Collection
Given that life-history traits such as body mass, generation length, and metabolic rates are linked to different aspects of molecular evolution (), it is plausible that convergent patterns of life-history evolution across extinction boundaries impart distinct signatures in the genomes of surviving lineages (10-12).For example, in plants, repeated evolution of polyploidy may be associated ...
Methods in Ecology and Evolution is an open access journal publishing papers across a wide range of subdisciplines, disseminating new methods in ecology and evolution. Abstract Sap flow measurements are fundamental to understanding water use in trees and could aid in predicting climate change effects on forest function.
The genetic diversity of B. pseudomallei is closely associated with the genomic plasticity of this organism. Our findings suggest that the type of genomic insertion fragments appears to be the primary source of genomic diversity in B. pseudomallei and can be utilized to identify strains with genetic diversity. Upon analyzing the WGS results of ...
Background Base editing is a powerful tool for artificial evolution to create allelic diversity and improve agronomic traits. However, the great evolutionary potential for every sgRNA target has been overlooked. And there is currently no high-throughput method for generating and characterizing as many changes in a single target as possible based on large mutant pools to permit rapid gene ...
HBV evolution and diversity. An important factor that makes hepatitis B difficult to cure is the complex degree of HBV mutation. HBV belongs to the Hepadnaviridae family, which includes five genera of viruses that can infect different types of hosts (Magnius et al., 2020; Dill et al., 2016; Debat and Ng, 2019; Hahn et al., 2015). ...
A wealth of research into the relationships among diversity, stability, and ecosystem functioning has been conducted in recent years (reviewed by Balvanera et al. 2006, Hooper et al. 2005). The ...
"The evolution of New York-style pizza is really about technology," says Mirarchi. This next-level pizza is cooked at a lower temperature, say 500 to 700 degrees, as opposed to 900 degrees or ...
In Criminal Minds, the BAU uses a profiling technique that comes from the study of epigenetics.; Criminal Minds: Evolution Season 2 focused more on hunting criminals than delving into their psyche
FUND FOR EDUCATION ABROAD OVERVIEWThe Fund for Education Abroad (FEA) was established in 2010 as a 501(c)3 non-profit organization to enable more American college students to study abroad. Applicants from groups underrepresented in study abroad are given preference, in an effort to make the demographics of U.S. undergraduates studying abroad reflect the rich diversity of the U.S. population.